Selecting appropriate Magnetic Nanoparticles for Hyperthermia
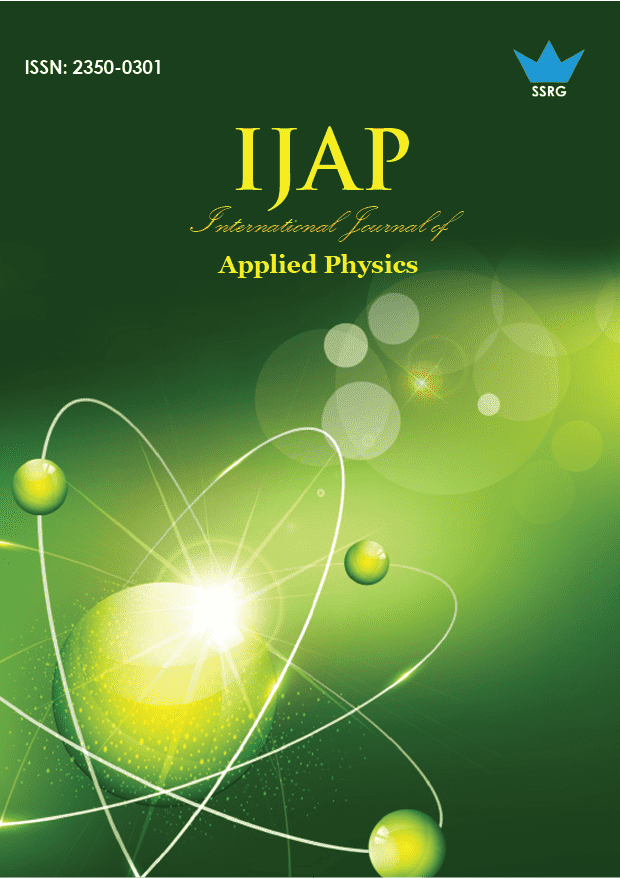
International Journal of Applied Physics |
© 2017 by SSRG - IJAP Journal |
Volume 4 Issue 2 |
Year of Publication : 2017 |
Authors : Hirakjyoti Das, Dambarudhar Mohanta, Pralay Kumar Karmakar, Himashri Das |
How to Cite?
Hirakjyoti Das, Dambarudhar Mohanta, Pralay Kumar Karmakar, Himashri Das, "Selecting appropriate Magnetic Nanoparticles for Hyperthermia," SSRG International Journal of Applied Physics, vol. 4, no. 2, pp. 11-15, 2017. Crossref, https://doi.org/10.14445/23500301/IJAP-V4I4P102
Abstract:
Due to immature cells, low blood flow rate, high density and lack of oxygen environment inside the tumour, it is experimentally seen that, tumour cells at temperature between 42°C and 46°C the viability of the cancerous cells is reduced (e.g. [5], [14]). Therefore efficiency of chemotherapy and radiation are increased. In recent years, due to the development in the area of nanotechnology, magnetic nanoparticle (MNPs) hyperthermia has been deeply studied as a promising new tumour therapy, because in presence of alternating magnetic field they show remarkable heating effects. In order to achieve efficient and safe operational hyperthermia conditions, it is necessary to study or investigate detail about what heating model or magnetic loss processes dominant over the other in the ensemble of nanoparticles which are injected at the cancerous tumour sites. Because there are more than one heat loss process involved in generating heat by MNPs. First experimental work on magnetic materials for hyperthermia was carried out by Gilchrist in 1957(e.g. [1]). He heated various tissue samples with the help of different sizes of γ -Fe2O3. Since then, there have numerous theoretical and experimental work been done by so many people on different nanoparticles. Here, in this work we compare theoretical results given by different MNPs. And taking into account cellular uptake mechanism it is showed that at a low frequency of applied magnetic field maghemite and at a high frequency of applied magnetic field FeCo are the best magnetic particle to use for the hyperthermia.
Keywords:
Magnetic Nanoparticles (MNPs), Nanoparticles (NPs), Relaxation time, Critical size, blocking temperature
References:
[1] Chaudhuri1, Giuseppe B. and R. Golestanian,2011. The effect of interactions on the cellular uptake of nanoparticles. IOP Science, 8 (2011) 046002 (9pp).
[2] Rudolf Hergt, Silvio Dutz, Robert Muller and Matthias Zeisberger.2016. Magnetic particle hyperthermia: nanoparticle magnetism and materials development for cancer therapy. J. Phys.: Condens. Matter 18 (2006) S2919–S2934.
[3] Silvio Dutz and Rudolf Hergt. 2014. Magnetic particle hyperthermia —a promising tumour therapy? Nanotechnology, 25 (2014) 452001 (28pp).
[4] Ihab M. Obaidat, Bashar Issa and Yousef Haik.2014. Magnetic Properties of Magnetic Nanoparticles for Efficient Hyperthermia. Nanomaterials 2015, 5, 63- 89; doi:10.3390/nano5010063.
[5] Andreas Jordan, Regina Scholz, Peter Wust, Horst FaK hling, Roland Felix.1999. Magnetic fluid hyperthermia (MFH): Cancer treatment with AC magnetic FIeld induced excitation of biocompatible superparamagnetic nanoparticles. Journal of Magnetism and Magnetic Materials 201 (1999) 413}419.
[6] S. Ruta, R. Chantrell1 & O. Hovorka.2015. Unified model of hyperthermia via hysteresis heating in systems of interacting magnetic nanoparticles. Scientific Reports.
[7] R. Hergta, R. Hiergeista, I. Hilgerb, W.A. Kaiserb, Y. Lapatnikovc, S. Margelc, U. Richterd. 2004. Maghemite nanoparticles with very high AC-losses for application in RF-magnetic hyperthermia. Journal of Magnetism and Magnetic Materials 270 (2004) 345–357.
[8] Robert J. Deissler,a) Yong Wu, and Michael A. Martens.2013. Dependence of Brownian and Néel relaxation times on magnetic field strength. Medical Physics 41, 012301 (2014); doi: 1118/1.4837216.
[9] Hui S Huang and James F Hainfeld.2013. Intravenous magnetic nanoparticle cancer hyperthermia. International Journal of Nanomedicine 2013:8 2521–2532.
[10] A. H. Habib, C. L. Ondeck, P. Chaudhary, M. R. Bockstaller, and M. E. McHenry.2008. Evaluation of iron-cobalt/ferrite core-shell nanoparticles for cancer thermotherapy. JOURNAL OF APPLIED PHYSICS 103, 07A307 _2008.
[11] Hamed Arami, R. M. Ferguson, Amit P. Khandhar, and Kannan M. Krishnan.2013. Size-dependent ferrohydrodynamic relaxometry of magnetic particle imaging tracers in different environments. Med Phys. 2013 Jul; 40(7): 071904.
[12] Ming Ma, Ya Wu, Jie Zhou, Yongkang Sun, Yu Zhang, Ning Gu.2002. Size dependence of specific power absorption of Fe3O4 particles in AC magnetic field. Journal of Magnetism and Magnetic Materials 268 (2004) 33–39.
[13] Vanchna Singh, Varsha Banerjee and Manish Sharma.2009. Dynamics of magnetic nanoparticle suspensions. J. Phys. D: Appl. Phys. 42 (2009) 245006 (9pp)
[14] C. L. Ondeck, A. H. Habib, P. Ohodnicki, K. Miller, C. A. Sawyer, P. Chaudhary, and M. E. McHenry. 2009. Theory of magnetic fluid heating with an alternating magnetic field with temperature dependent materials properties for self-regulated heating. JOURNAL OF APPLIED PHYSICS 105, 07B324 _2009.
[15] Riadh W. Y. Habash, Rajeev Bansal, Daniel Krewski, and Hafid T. Alhafid4.2006. Thermal Therapy, Part 2: Hyperthermia Techniques. Biomedical Engineering, 34(6):491–542 a(2006).
[16] Karolin Franke, Melanie Kettering, Kathleen Lange, Werner A Kaiser, Ingrid Hilger. 2013. The exposure of cancer cells to hyperthermia, iron oxide nanoparticles, and mitomycin C influences membrane multidrug resistance protein expression levels. International Journal of Nanomedicine 2013:8 351–363.
[17] Irene Andreu and Eva Natividad.2013. Accuracy of available methods for quantifying the heat power generation of nanoparticles for magnetic hyperthermia. Int J Hyperthermia, 2013; 29(8): 739– 751.
[18] W. Andra, C.G. dÕAmbly, R. Hergt, I. Hilger, W.A. Kaiser.1999. Temperature distribution as function of time around a small spherical heat source of local magnetic hyperthermia. Journal of Magnetism and Magnetic Materials 194 (1999) 197-203.