Home Rehabilitation of Patients using Bioelectronics Nano-Medicine Devices and 65nm CMOS Embedded Electronics for Insulation to Avoid Coronavirus Infection
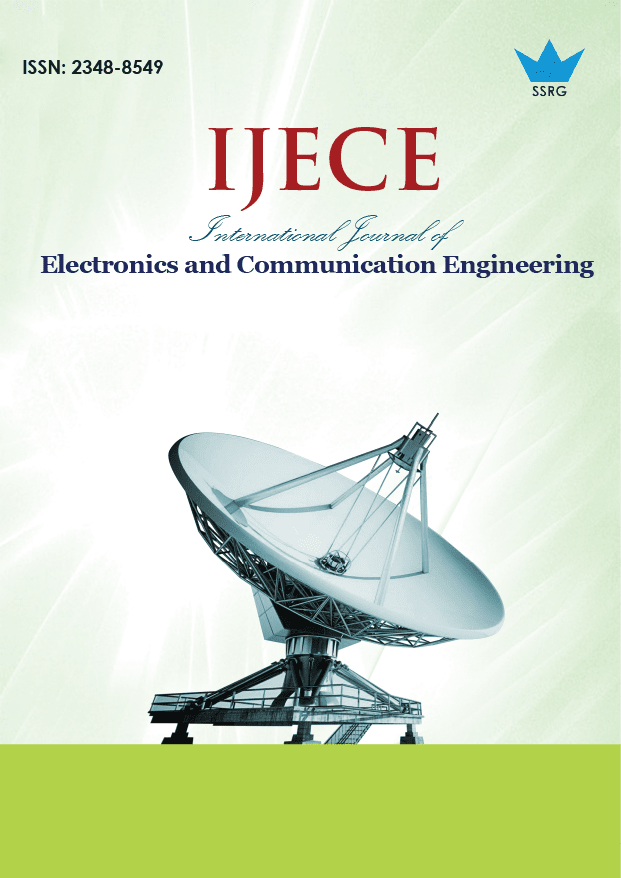
International Journal of Electronics and Communication Engineering |
© 2022 by SSRG - IJECE Journal |
Volume 9 Issue 4 |
Year of Publication : 2022 |
Authors : Hafez Fouad, Hesham Kamel |
How to Cite?
Hafez Fouad, Hesham Kamel, "Home Rehabilitation of Patients using Bioelectronics Nano-Medicine Devices and 65nm CMOS Embedded Electronics for Insulation to Avoid Coronavirus Infection," SSRG International Journal of Electronics and Communication Engineering, vol. 9, no. 4, pp. 1-9, 2022. Crossref, https://doi.org/10.14445/23488549/IJECE-V9I4P101
Abstract:
This paper aims to adopt nano-medicine devices with bionics (function like living organisms) in embedded systems (isolation) to allow health rehabilitation of patients at home to avoid infection with the Coronavirus. Measuring various patient's vital signals such as temperature, blood oxygen percentage, blood pressure, heartbeat, and glucose level (to pump insulin to the body to control the blood sugar level) are included in our objective. In this work, we developed a Class AB 65nm CMOS system that can be used as part of Medical IOT applications in Telemedicine and remote healthcare. Our proposed circuit has been designed and simulated using CADENCE Design Tools in TMSC 65nm CMOS technology to optimize the design and get the required specifications. This paper designed a 2.4 GHz Class AB CMOS PA with a CG driver stage followed by a CS power stage using the proposed method for WBAN applications. The simulation results showed that the proposed PA achieved a maximum PAE of 49.09% and had an average gain of 32.4 dB over the frequency of interest in Industrial, Scientific, and Medical (ISM) bands. Input Reflection Coefficient S11=-38.588 dB, Psat=15.6172 dBm Output referred compression point P1dB=11.784 dBm. The Physical layout of the proposed CMOS Class-AB Amplifier has been designed and optimized to a 0.085mm2 chip area.
Keywords:
Bioelectronics, Wearable and Implantable sensors, Power amplifier, Class AB, Embedded systems, Healthcare monitoring, nano-medicine, Coronavirus, Internet of Things IoT, 65nm CMOS, Energy harvest, Organic devices.
References:
[1] George D. Spyropoulos et al., “Mixed-Conducting Particulate Composites for Soft Electronics,” Science Advances, vol. 6, no. 17, 2020. Crossref, http://doi.org/10.1126/sciadv.aaz6767
[2] Eleonora Macchia et al., “About the Amplification Factors in Organic Bioelectronic Sensors,” The Royal Society of Chemistry, vol. 7, pp. 999-1013, 2020. Crossref, http://doi.org/10.1039/c9mh01544b
[3] Ariana Villarroel Marquez, Niall McEvoy, and Amir Pakdel, “Organic Electrochemical Transistors (OECTs) toward Flexible and Wearable Bioelectronics,” Journal of Molecules, Department of Mechanical, Manufacturing & Biomedical Engineering, vol. 25, no. 22, p. 5288, 2020. Crossref, https://doi.org/10.3390/molecules25225288
[4] Meenakshi et al., “Switchable Graphene-Based Bioelectronics Interfaces,” Chemosensors, vol. 8, no. 2, pp. 45, 2020. Crossref, https://doi.org/10.3390/chemosensors8020045
[5] Godspower W. Omokhunu, and Christian Bach, “Organic Bio-Electronics: Bridging the Gap Between Natural and Artificial Materials for Bio-Electronics,” EJERS European Journal of Engineering Research and Science, vol. 4, no. 1, pp. 85-91, 2019. Crossref, https://doi.org/10.24018/ejers.2019.4.1.635
[6] Krishna Feron et al., “Organic Bioelectronics: Materials and Biocompatibility,” International Journal of Molecular Sciences, License CC BY, vol. 19, no. 8, pp. 2382, 2018. Crossref, https://doi.org/10.3390/ijms19082382
[7] Hyun SeokSong et al., “3D Hydrogel Scaffold Doped with 2D Graphene Materials for Biosensors and Bioelectronics,” Biosensors and Bioelectronics, vol. 89, no. 1, pp. 187-200, 2017. Crossref, https://doi.org/10.1016/j.bios.2016.03.045
[8] Yoichi Aoki, “Photovoltaic Performance of Organic Photovoltaics for Indoor Energy Harvester,” Organic Electronics, vol. 48, no. c, pp. 194-197, 2017. Crossref, https://doi.org/10.1016/j.orgel.2017.05.023
[9] D. R. Cox et al., Organic Photovoltaic Cells: Heliatek Claims Conversion Efficiency Record by Nancy Owano TechXplore.com, Statistical Thinking for 21st-Century Scientists, Science Advances, 2017.
[10] Seth R Marder, and Jean-Luc Bredas, “The WSPC Reference on Organic Electronics: Organic Semiconductors, Fundamental Aspects of Materials and Applications,” Saudi Arabia & Georgia Institute of Technology, King Abdullah University of Science & Technology, USA, vol. 2, no. 2, 2016. Crossref, https://doi.org/10.1142/9678.
[11] Srilalitha, Jayaveera, and Madhvendhra, “The Effect of Dopant, Temperature, and Band Gap on Conductivity of Conducting Polymers,” International Journal of Innovative Research in Science, Engineering and Technology, vol. 2, no. 7, 2013.
[12] Kareema Majeed Ziadan, Conducting Polymers Application, New Polymers for Special Application, Intech, 2012.
[13] Susanne Löffler, Ben Libberton, and Agneta Richter-Dahlfors, “Organic Bioelectronic Tools for Biomedical Applications,” Electronics, vol. 4, no. 4, pp. 879-908, 2015. Crossref, https://doi.org/10.3390/electronics4040879
[14] Alwin Ming-Doug Wan et al., “3D Conducting Polymer Platforms for Electrical Control of Protein Conformation and Cellular Functions,” Journal of Materials Chemistry B, vol. 3, pp. 5040-5048, 2015. Crossref, https://doi.org/10.1039/C5TB00390C
[15] Mawad D, Lauto A, and Wallace G.G, “Polymeric Hydrogels as Smart Biomaterials,” Kalia, S., Ed., Springer Series on Polymer and Composite Materials; Springer: Cham, Switzerland, 2016.
[16] Mohammad Javadi et al., “Conductive Tough Hydrogel for Bioapplications,” Macromolecular Bioscience, vol. 18, no. 2, 2017. Crossref, https://doi.org/10.1002/mabi.201700270
[17] G.L.Mario Cheong et al., “Conductive Hydrogels with Tailored Bioactivity for Implantable Electrode Coatings,” Acta Biomaterials, vol. 10, no. 3, pp. 1216-1226, 2014. Crossref, https://doi.org/10.1016/j.actbio.2013.12.032
[18] Anton Mihic et al., “A Conductive Polymer Hydrogel Supports Electrical Cell Signaling and Improves Cardiac Function After Implantation Into a Myocardial Infarct,” Circulation, vol. 132, no. 8, pp. 772-784, 2015. Crossref, https://doi.org/10.1161/CIRCULATIONAHA.114.014937
[19] Josef Goding et al., “A Living Electrode Construct for Incorporating Cells Into Bionic Devices,” MRS Communication, vol. 7, no. 3, pp. 487-495, 2017. Crossref, https://doi.org/10.1557/mrc.2017.44
[20] Baolin Guo, Lidija Glavas, and Ann-Christine Albertsson, “Biodegradable and Electrically Conducting Polymers for Biomedical Applications,” Progress in Polymer Science, vol. 38, no. 9, pp. 1263-1286, 2013. Crossref, https://doi.org/10.1016/j.progpolymsci.2013.06.003Get rights and content
[21] Amin S. Ibrahim et al., “Traffic Modeling of Smart City Internet of Things Architecture,” IET Communication, vol. 14, no. 8, pp. 1275-1284, 2020. Crossref, https://doi.org/10.1049/iet-com.2019.1252
[22] Ericsson Mobility Report, 2021. [Online]. Available: https://www.ericsson.com/en/reports-and-papers/mobility-report/reports/november-2021
[23] [Online]. Available: https://www.extremenetworks.com/wifi6/what-is-80211ax/
[24] “ITU Radio Regulations Articles,” Edition, 2020.
[25] “Technology Strategic Guidance Spectrum Optimization,” VEON Group, 2013.