Performance Analysis of Bismuth-Based CsBi3I10 Tandem Perovskite Solar Cell
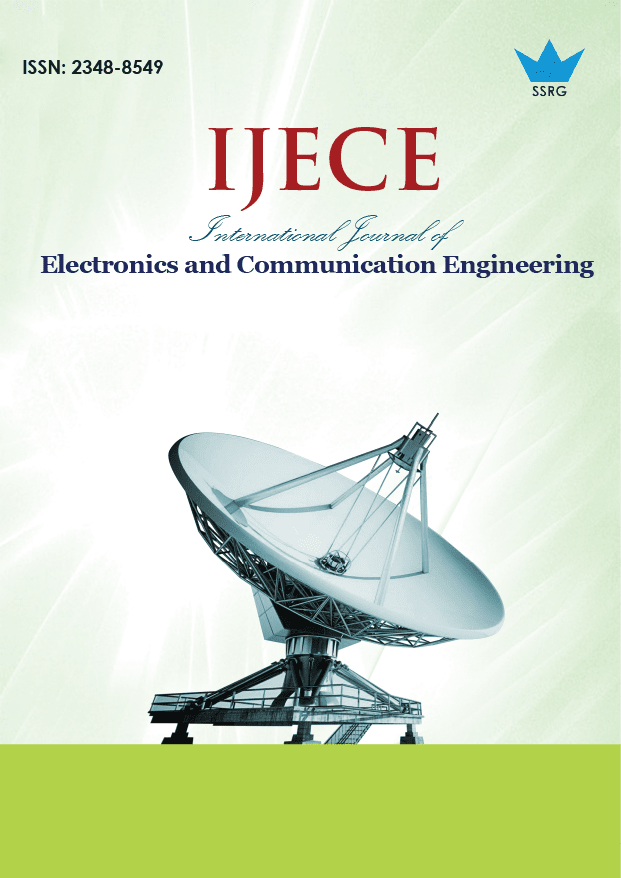
International Journal of Electronics and Communication Engineering |
© 2024 by SSRG - IJECE Journal |
Volume 11 Issue 4 |
Year of Publication : 2024 |
Authors : Shreyus Goutham Kumar, H.G. Abhigna, N. Sindhu, C.R. Prashanth |
How to Cite?
Shreyus Goutham Kumar, H.G. Abhigna, N. Sindhu, C.R. Prashanth, "Performance Analysis of Bismuth-Based CsBi3I10 Tandem Perovskite Solar Cell," SSRG International Journal of Electronics and Communication Engineering, vol. 11, no. 4, pp. 121-132, 2024. Crossref, https://doi.org/10.14445/23488549/IJECE-V11I4P113
Abstract:
With the ever-increasing energy demands, the quest for renewable energy has led to the exploration of inorganic perovskite solar cells having a higher efficiency of more than 25.5%. The full solar spectrum is exploited to achieve a higher power conversion efficiency to surpass the Shockley-Queisser limit. Using SCAPS-1D, this work simulates single and tandem solar cells. This simulation features a CsBi3I10 perovskite absorber layer sandwiched between the electron transport layer (ETL) and hole transport layer (HTL). The standalone cell achieved a Power Conversion Efficiency (PCE) of 21.6%, a fill factor of 82.2%, Jsc of 20.9 mA/cm2 , and Voc of 1.25 V. To bypass the Shockley-Queisser limit, the solar cell is integrated into the crystalline silicon solar cell by mechanically stacking on top of each other. Tandem cells achieved a power conversion efficiency of 30.8%, higher than a single cell. The paper discusses the impact of choosing different combinations of ETL and HTL layers. According to the simulation, CuSbS2 and SnO2 are the best combinations for HTL and ETL. Apart from single and tandem cell performance analysis, the paper explores the effect of the absorber layer’s thickness, bandgap, and defect density, along with ETL and HTL thickness. A Tandem solar cell with CsBi3I10 and the efficiency by the tandem configuration is 30.8% (FF=77.69%, Jsc=13.5 mA/cm2 , Voc=1.77 V).
Keywords:
Perovskite solar cells, Tandem devices, SCAPS 1-D, Power conversion efficiency, Lead-free, Fill-Factor.
References:
[1] Bas J. Van Ruijven, Enrica De Cian, and Ian Sue Wing, “Amplification of Future Energy Demand Growth Due to Climate Change,” Nature Communications, pp. 1-12, 2019.
[CrossRef] [Google Scholar] [Publisher Link]
[2] L.L. Yan et al., “A Review on the Crystalline Silicon Bottom Cell for Monolithic Perovskite/Silicon Tandem Solar Cells,” Materials Today Nano, vol. 7, 2019.
[CrossRef] [Google Scholar] [Publisher Link]
[3] Tomas Leijtens et al., “Opportunities and Challenges for Tandem Solar Cells Using Metal Halide Perovskite Semiconductors,” Nature Energy, pp. 828-838, 2018.
[CrossRef] [Google Scholar] [Publisher Link]
[4] Kihwan Kim et al., “Simulations of Chalcopyrite/c-Si Tandem Cells Using SCAPS-1D,” Solar Energy, vol. 145, pp. 52-58, 2017.
[CrossRef] [Google Scholar] [Publisher Link]
[5] Usha Mandadapu, S. Victor Vedanayakam, and K. Thyagarajan, “Simulation and Analysis of Lead Based Perovskite Solar Cell Using SCAPS-1D,” Indian Journal of Science and Technology, vol. 10, no. 11, pp. 1-8, 2017.
[Google Scholar] [Publisher Link]
[6] Jenny A. Nelson, The Physics of Solar Cells, World Scientific Publishing Company, pp. 1-384, 2003.
[Google Scholar] [Publisher Link]
[7] Simon M. Sze, and Kwok K. Ng, Physics of Semiconductor Devices, Wiley, pp. 1-832, 2006.
[Google Scholar] [Publisher Link]
[8] Peter Y. Yu, and Manuel Cardona, Fundamentals of Semiconductors : Physics and Materials Properties, Springer Berlin, Heidelberg, pp. 1-617, 1996.
[Google Scholar] [Publisher Link]
[9] Martin A. Green, Solar Cells Operating Principles, Technology, and System Applications, Prentice-Hall, pp. 1-274, 1992.
[Google Scholar] [Publisher Link]
[10] Shamim Ahmmed et al., “Performance Analysis of lead-Free CsBi3I10-Based Perovskite Solar Cell through the Numerical Calculation,” Solar Energy, vol. 226, pp. 54-63, 2021.
[CrossRef] [Google Scholar] [Publisher Link]
[11] Bingjuan Zhang et al., “NiO/Perovskite Heterojunction Contact Engineering for Highly Efficient and Stable Perovskite Solar Cells,” Advanced Science, vol. 7, no. 11, pp. 1-10, 2020.
[CrossRef] [Google Scholar] [Publisher Link]
[12] Pandiyarajan Mariyappan et al., “Influence of Inorganic NiOx Hole Transport Layer on the Growth of CsBi3I10 Perovskite Films for Photovoltaic Applications,” Advanced Materials Interfaces, vol. 8, no. 7, 2021.
[CrossRef] [Google Scholar] [Publisher Link]
[13] I. Chabri et al., “Numerical Analysis of Lead-Free Cs2SnI6-Based Perovskite Solar Cell, with Inorganic Charge Transport Layers Using SCAPS-1D” Journal of Electronic Materials, vol. 52, pp. 2722-2736, 2023.
[CrossRef] [Google Scholar] [Publisher Link]
[14] Faiza Azri et al., “Electron and Hole Transport Layers Optimization by Numerical Simulation of a Perovskite Solar Cell,” Solar Energy, vol. 181, pp. 372-378, 2019.
[CrossRef] [Google Scholar] [Publisher Link]
[15] Jian Kang et al., “Alloying Sb into All Inorganic Lead-Free CsBi3I10 for Improving the Crystal Growth and Photovoltaic Performance,” Journal of Materials Chemistry A, vol. 10, no. 37, pp. 19618-19625, 2022.
[CrossRef] [Google Scholar] [Publisher Link]
[16] M. Khalid Hossain et al., “Combined DFT, SCAPS-1D, and wxAMPS Frameworks for Design Optimization of Efficient Cs2BiAgI6- Based Perovskite Solar Cells with Different Charge Transport Layers,” RSC Advances, vol. 12, no. 54, pp. 34850-34873, 2022.
[CrossRef] [Google Scholar] [Publisher Link]
[17] M. Khalid Hossain et al., “Effect of Various Electron and Hole Transport Layers on the Performance of CsPbI3-Based Perovskite Solar Cells: A Numerical Investigation in DFT, SCAPS-1D, and wxAMPS Frameworks,” ACS Omega, vol. 7, no. 47, pp. 43210-43230, 2022.
[CrossRef] [Google Scholar] [Publisher Link]
[18] Lingyan Lin et al., “A Modeled Perovskite Solar Cell Structure with a Cu2O Hole-Transporting Layer Enabling Over 20% Efficiency by Low-Cost Low-Temperature Processing,” Journal of Physics and Chemistry of Solids, vol. 124, pp. 205-211, 2019.
[CrossRef] [Google Scholar] [Publisher Link]
[19] Lu-Lu Jiang et al., “Interface Engineering Toward Enhanced Efficiency of Planar Perovskite Solar Cells,” Journal of Materials Chemistry A, vol. 4, no. 1, pp. 217-222, 2016.
[CrossRef] [Google Scholar] [Publisher Link]
[20] Zhanglin Guo et al., “High Electrical Conductivity 2D MXene Serves as Additive of Perovskite for Efficient Solar Cells,” Small, vol. 14, no. 47, 2018.
[CrossRef] [Google Scholar] [Publisher Link]
[21] Marwa Sayed Salem Basyoni et al., “On the Investigation of Interface Defects of Solar Cells: Lead-Based vs Lead-Free Perovskite,” IEEE Access, vol. 9, pp. 130221-130232, 2021.
[CrossRef] [Google Scholar] [Publisher Link]
[22] Shambhavi Rai, B.K. Pandey, and D.K. Dwivedi, “Modeling of Highly Efficient and Low Cost CH3NH3Pb(I1-xClx)3 Based Perovskite Solar Cell by Numerical Simulation,” Optical Materials, vol. 100, 2020.
[CrossRef] [Google Scholar] [Publisher Link]
[23] Aminreza Mohandes, Mahmood Moradi, and Hamid Nadgaran, “Numerical Simulation of Inorganic Cs2AgBiBr6 as a Lead-Free Perovskite Using Device Simulation SCAPS-1D,” Optical and Quantum Electronics, vol. 53, 2021.
[CrossRef] [Google Scholar] [Publisher Link]
[24] C. Ulbrich et al., “Matching of Silicon Thin-Film Tandem Solar Cells for Maximum Power Output,” International Journal of Photoenergy, vol. 2013, pp. 1-7, 2013.
[CrossRef] [Google Scholar] [Publisher Link]
[25] M. Bonnet-Eymard et al., “Current Matching Optimization in High-Efficiency Thin-Film Silicon Tandem Solar Cells,” 2013 IEEE 39th Photovoltaic Specialists Conference (PVSC), Tampa, FL, USA, pp. 184-187, 2013.
[CrossRef] [Google Scholar] [Publisher Link]