MEMS-Based Biosensors for Mass Detection in Single Cells-Review of Techniques and Approaches
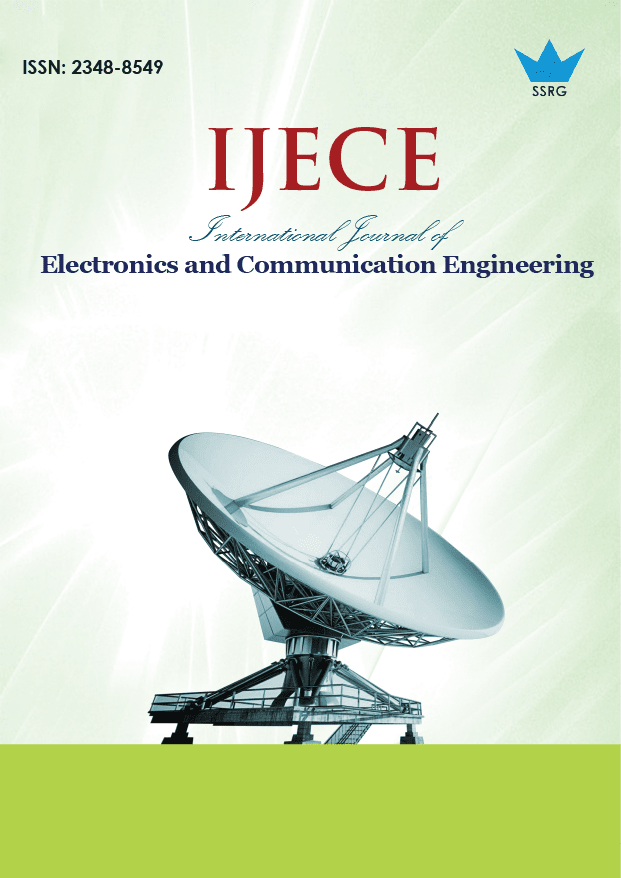
International Journal of Electronics and Communication Engineering |
© 2024 by SSRG - IJECE Journal |
Volume 11 Issue 8 |
Year of Publication : 2024 |
Authors : A.L.G.N. Aditya, Elizabeth Rufus |
How to Cite?
A.L.G.N. Aditya, Elizabeth Rufus, "MEMS-Based Biosensors for Mass Detection in Single Cells-Review of Techniques and Approaches," SSRG International Journal of Electronics and Communication Engineering, vol. 11, no. 8, pp. 312-324, 2024. Crossref, https://doi.org/10.14445/23488549/IJECE-V11I8P130
Abstract:
The significance of early detection and diagnosis in medicine is widely acknowledged, whereas clinicopathological methods, which are more effective after diseases have progressed, tend to receive less attention. With hematological diseases, the chances of cure or extension of life span are higher with early-onset diagnosis. Early detection of the disease onset and progression is vital and challenging for reasons of minuscule changes in molecular or cellular behaviors. Cells are the basic building block, so understanding the changes at the cellular level is crucial to estimating the ecological and biogeochemical models. In the case of single-cell studies, sensitivity and resolution are the key challenges that the scientific community addresses as the key focus areas. With traditional label-free sensing techniques, though these challenges are addressed, the sample preparation, handling, and characterization are setbacks for making their gold standards. With emerging technologies incidental to micro/nanofabrication processes, MEMS-based resonant sensors emerged as a paradigm technique for the detection of biophysical changes in a single-cell study due to their good sensitivity and resolution. This paper focuses on the review of techniques and methods to detect the mass of biological cells and compares their results with the MEMS Resonating mass sensors. It also focuses on the technique of resonant frequency shift and approaches for improving the sensitivity of the resonators by focusing on reported structures.
Keywords:
Cell behaviour studies, Label-free sensing, Micro/nano-fabrication, Micro-electro mechanical system, Resonant sensors.
References:
[1] David M. Eddy, and Charles H. Clanton, “The Art of Diagnosis — Solving the Clinicopathological Exercise,” The Central African Journal of Medicine, vol. 306, no. 21, pp. 1263-1268, 1982.
[CrossRef] [Google Scholar] [Publisher Link]
[2] Momoko Hamano et al., “Prediction of Single-Cell Mechanisms for Disease Progression in Hypertrophic Remodelling by a Trans-Omics Approach,” Scientific Reports, vol. 11, pp. 1-17, 2021.
[CrossRef] [Google Scholar] [Publisher Link]
[3] Seitaro Nomura, “Single-Cell Genomics to Understand Disease Pathogenesis,” Journal of Human Genetics, vol. 66, pp. 75-84, 2021.
[CrossRef] [Google Scholar] [Publisher Link]
[4] Nathan Cermak et al., “High-Throughput Measurement of Single-Cell Growth Rates Using Serial Microfluidic Mass Sensor Arrays,” Nature Biotechnology, vol. 34, pp. 1052-1059, 2016.
[CrossRef] [Google Scholar] [Publisher Link]
[5] Thomas P. Burg, “Devices with Embedded Channels,” Advanced Micro and Nanosystems, 2015.
[CrossRef] [Google Scholar] [Publisher Link]
[6] Sungmin Son et al., “Direct Observation of Mammalian Cell Growth and Size Regulation,” Nature Methods, vol. 9, pp. 910-912, 2012.
[CrossRef] [Google Scholar] [Publisher Link]
[7] Alexey Mozharov et al., “Nanomass Sensing Via Node Shift Tracing in Vibrations of Coupled Nanowires Enhanced by Fano Resonances,” ACS Applied Nano Materials, vol. 4, no. 11, pp. 11989-11996, 2021.
[CrossRef] [Google Scholar] [Publisher Link]
[8] Nathan Cermak et al., “Direct Single-Cell Biomass Estimates for Marine Bacteria Via Archimedes’ Principle,” The ISME Journal, vol. 11, no. 3, pp. 825-828, 2016.
[CrossRef] [Google Scholar] [Publisher Link]
[9] T.P. Burg, and S.R. Manalis, “Suspended Microchannel Resonators for Biomolecular Detection,” Applied Physics Letters, vol. 83, no. 13, pp. 2698-2700, 2003.
[CrossRef] [Google Scholar] [Publisher Link]
[10] Kidong Park et al., “Measurement of Adherent Cell Mass and Growth,” Proceedings of the National Academy of Sciences of the United States of America, vol. 107, no. 48, pp. 20691-20696, 2010.
[CrossRef] [Google Scholar] [Publisher Link]
[11] Paul Jorgensen, and Mike Tyers, “How Cells Coordinate Growth and Division,” Current Biology, vol. 14, no. 23, pp. 1014-1027, 2004.
[CrossRef] [Google Scholar] [Publisher Link]
[12] Sung-Jin Park et al., “Piezoresistive Cantilever Performance-Part II: Optimization,” Journal of Microelectromechanical Systems, vol. 19, no. 1, pp. 149-161, 2010.
[CrossRef] [Google Scholar] [Publisher Link]
[13] Amit Gupta, Demir Akin, and Rashid Bashir, “Detection of Bacterial Cells and Antibodies Using Surface Micromachined Thin Silicon Cantilever Resonators,” Journal of Vacuum Science & Technology B: Microelectronics and Nanometer Structures Processing, Measurement, and Phenomena, vol. 22, pp. 2785-2791, 2004.
[CrossRef] [Google Scholar] [Publisher Link]
[14] Angelica P. Davila et al., “Microresonator Mass Sensors for Detection of Bacillus Anthracis Sterne Spores in Air and Water,” Biosensors and Bioelectronics, vol. 22, no. 12, pp. 3028-3035, 2007.
[CrossRef] [Google Scholar] [Publisher Link]
[15] A. Gupta, D. Akin, and R. Bashir, “Single Virus Particle Mass Detection Using Microresonators with Nanoscale Thickness,” Applied Physics Letters, vol. 84, pp. 1976-1978, 2004.
[CrossRef] [Google Scholar] [Publisher Link]
[16] Karin Y. Gfeller, Natalia Nugaeva, and Martin Hegner, “Micromechanical Oscillators as Rapid Biosensor for the Detection of Active Growth of Escherichia Coli,” Biosensors and Bioelectronics, vol. 21, no. 3, pp. 528-533, 2005.
[CrossRef] [Google Scholar] [Publisher Link]
[17] K. Park et al., “MEMS Mass Sensors with Uniform Sensitivity for Monitoring Cellular Apoptosis,” 16th International Solid-State Sensors, Actuators and Microsystems Conference, Beijing, China, pp. 759-762, 2011.
[CrossRef] [Google Scholar] [Publisher Link]
[18] Lawrence W. Bergman, “Growth and Maintenance of Yeast,” Two-Hybrid Systems, vol. 177, pp. 9-14, 2001.
[CrossRef] [Google Scholar] [Publisher Link]
[19] Mansooreh Ahmadian et al., “A Hybrid Stochastic Model of the Budding Yeast Cell Cycle,” Npj Systems Biology and Applications, vol. 6, pp. 1-10, 2020.
[CrossRef] [Google Scholar] [Publisher Link]
[20] George I. Bell, and Ernest C. Anderson, “Cell Growth and Division a Mathematical Model with Applications to Cell Volume Distributions,” Biophysical Journal, vol. 7, no. 4, pp. 329-351, 1967.
[CrossRef] [Google Scholar] [Publisher Link]
[21] Daniel A. Charlebois, and Gábora Balázsi, “Modeling Cell Population Dynamics,” Silico Biology, vol. 13, pp. 21-39, 2019.
[CrossRef] [Google Scholar] [Publisher Link]
[22] Joseph J. Crivelli et al., “A Mathematical Model for Cell Cycle-Specific Cancer Virotherapy,” Journal of Biological Dynamics, vol. 6, pp. 104-120, 2012.
[CrossRef] [Google Scholar] [Publisher Link]
[23] Frederick R. Cross et al., “Testing a Mathematical Model of the Yeast Cell Cycle,” Molecular Biology of the Cell, vol. 13, no. 1, pp. 52- 70, 2002.
[CrossRef] [Google Scholar] [Publisher Link]
[24] Katarzyna A. Rejniak, “A Single-Cell Approach in Modeling the Dynamics of Tumor Microregions,” Mathematical Biosciences and Engineering, vol. 2, no. 3, pp. 643-655, 2005.
[CrossRef] [Google Scholar] [Publisher Link]
[25] Rahul Singhvi et al., “Engineering Cell Shape and Function,” Science, vol. 264, no. 5159, pp. 696-698, 2014.
[CrossRef] [Google Scholar] [Publisher Link]
[26] Katherine C. Chen et al., “Integrative Analysis of Cell Cycle Control in Budding Yeast,” Molecular Biology of the Cell, vol. 15, no. 8, pp. 3841-3862, 2004.
[CrossRef] [Google Scholar] [Publisher Link]
[27] Kaiqun Lin et al., “Surface Plasmon Resonance Hydrogen Sensor Based on Metallic Grating with High Sensitivity,” Optics Express, vol. 16, no. 23, pp. 18599-18604, 2008.
[CrossRef] [Google Scholar] [Publisher Link]
[28] Mary Pickering et al., “Fission Yeast Cells Grow Approximately Exponentially,” Cell Cycle, vol. 18, no. 8, pp. 869-879, 2019.
[CrossRef] [Google Scholar] [Publisher Link]
[29] Ilya Soifer, and Naama Barkai, “Systematic Identification of Cell Size Regulators in Budding Yeast,” Molecular Systems Biology, vol. 10, pp. 1-15, 2014.
[CrossRef] [Google Scholar] [Publisher Link]
[30] Alessandro D. Trigilio et al., “Gillespie-Driven kinetic Monte Carlo Algorithms to Model Events for Bulk or Solution (Bio)Chemical Systems Containing Elemental and Distributed Species,” Industrial & Engineering Chemistry Research, vol. 59, no. 41, pp. 18357-18386, 2020.
[CrossRef] [Google Scholar] [Publisher Link]
[31] Jie Wu, “Biased AC Electro-Osmosis for On-Chip Bioparticle Processing,” IEEE Transactions on Nanotechnology, vol. 5, no. 2, pp. 84- 89, 2006.
[CrossRef] [Google Scholar] [Publisher Link]
[32] Jiang Zhe et al., “A Micromachined High Throughput Coulter Counter for Bioparticle Detection and Counting,” Journal of Micromechanics and Microengineering, vol. 17, no. 2, pp. 304-313, 2007.
[CrossRef] [Google Scholar] [Publisher Link]
[33] J. Lu, and Z. Rosenzweig, “Nanoscale Fluorescent Sensors for Intracellular Analysis,” Fresenius’ Journal of Analytical Chemistry, vol. 366, pp. 569-575, 2000.
[CrossRef] [Google Scholar] [Publisher Link]
[34] Rosario Rizzuto, Walter Carrington, and Richard A Tuft, “Digital Imaging Microscopy of Living Cells,” Trends in Cell Biology, vol. 8, no. 7, pp. 288-292, 1998.
[CrossRef] [Google Scholar] [Publisher Link]
[35] Xiao-Hong Xu, and Edward S. Yeung, “Direct Measurement of Single-Molecule Diffusion and Photodecomposition in Free Solution,” Science, vol. 275, no. 5303, pp. 1106-1109, 1997.
[CrossRef] [Google Scholar] [Publisher Link]
[36] Ilkka Ojanperä, Marjo Kolmonen, and Anna Pelander, “Current Use of High-Resolution Mass Spectrometry in Drug Screening Relevant to Clinical and Forensic Toxicology and Doping Control,” Analytical and Bioanalytical Chemistry, vol. 403, no. 5, pp. 1203-1220, 2012.
[CrossRef] [Google Scholar] [Publisher Link]
[37] Michelle Wood et al., “Recent Applications of Liquid Chromatography-Mass Spectrometry in Forensic Science,” Journal of Chromatography A, vol. 1130, no. 1, pp. 3-15, 2006.
[CrossRef] [Google Scholar] [Publisher Link]
[38] R.J.B. Peters et al., “Screening in Veterinary Drug Analysis and Sports Doping Control Based on Full-Scan, Accurate-Mass Spectrometry,” TrAC - Trends in Analytical Chemistry, vol. 29, no. 11, pp. 1250-1268, 2010.
[CrossRef] [Google Scholar] [Publisher Link]
[39] Heng-Hui Gan, Christos Soukoulis, and Ian Fisk, “Atmospheric Pressure Chemical Ionisation Mass Spectrometry Analysis Linked with Chemometrics for Food Classification - A Case Study: Geographical Provenance and Cultivar Classification of Monovarietal Clarified Apple Juices,” Food Chemistry, vol. 146, pp. 149-156, 2014.
[CrossRef] [Google Scholar] [Publisher Link]
[40] Lukas Vaclavik et al., “Liquid Chromatography-Mass Spectrometry-Based Metabolomics for Authenticity Assessment of Fruit Juices,” Metabolomics, vol. 8, pp. 793-803, 2012.
[CrossRef] [Google Scholar] [Publisher Link]
[41] Gérard Hopfgartner, David Tonoli, and Emmanuel Varesio, “High-Resolution Mass Spectrometry for Integrated Qualitative and Quantitative Analysis of Pharmaceuticals in Biological Matrices,” Analytical and Bioanalytical Chemistry, vol. 402, pp. 2587-2596, 2012.
[CrossRef] [Google Scholar] [Publisher Link]
[42] Hanan Awad, Mona M. Khamis, and Anas El-Aneed, “Mass Spectrometry, Review of the Basics: Ionization,” Applied Spectroscopy Reviews, vol. 50, no. 2, pp. 158-175, 2015.
[CrossRef] [Google Scholar] [Publisher Link]
[43] Patrick C. Chaumet et al., “Quantitative Phase Microscopies: Accuracy Comparison,” Arxiv, pp. 1-36, 2024.
[CrossRef] [Google Scholar] [Publisher Link]
[44] Thang L. Nguyen et al., “Quantitative Phase Imaging: Recent Advances and Expanding Potential in Biomedicine,” ACS Nano, vol. 16, no. 8, pp. 11516-11544, 2022.
[CrossRef] [Google Scholar] [Publisher Link]
[45] J.G. Gordon, and S. Ernst, “Surface Plasmons as a Probe of the Electrochemical Interface,” Surface Science, vol. 101, no. 1-3, pp. 499- 506, 1980.
[CrossRef] [Google Scholar] [Publisher Link]
[46] Stefan Löfås et al., “Bioanalysis with Surface Plasmon Resonance,” Sensors and Actuators: B. Chemical, vol. 5, no. 1-4, pp. 79-84, 1991.
[CrossRef] [Google Scholar] [Publisher Link]
[47] Barbora Špačková et al., “Optical Biosensors Based on Plasmonic Nanostructures: A Review,” Proceedings of the IEEE, vol. 104, no. 12, pp. 2380-2408, 2016.
[CrossRef] [Google Scholar] [Publisher Link]
[48] Tobias Maier, Marc Güell, and Luis Serrano, “Correlation of mRNA and Protein in Complex Biological Samples,” FEBS Letters, vol. 583, no. 24, pp. 3966-3973, 2009.
[CrossRef] [Google Scholar] [Publisher Link]
[49] Barbora Špačková et al., “Nanoplasmonic–Nanofluidic Single-Molecule Biosensors for Ultrasmall Sample Volumes,” ACS Sensors, vol. 6, no. 1, pp. 73-82, 2021.
[CrossRef] [Google Scholar] [Publisher Link]
[50] L. Streppa et al., “Tracking in Real Time the Crawling Dynamics of Adherent Living Cells with a High-Resolution Surface Plasmon Microscope,” Plasmonics in Biology and Medicine XIII, vol. 9724, pp. 1-10, 2016.
[CrossRef] [Google Scholar] [Publisher Link]
[51] B. Ilic, “Attogram Detection Using Nanoelectromechanical Oscillators,” Journal of Applied Physics, vol. 95, pp. 3674-3703, 2004.
[CrossRef] [Google Scholar] [Publisher Link]
[52] Thomas P. Burg et al., “Vacuum-Packaged Suspended Microchannel Resonant Mass Sensor for Biomolecular Detection,” Journal of Microelectromechanical Systems, vol. 15, no. 6, pp. 1466-1476, 2006.
[CrossRef] [Google Scholar] [Publisher Link]
[53] T.P. Burg, and S.R. Manalis, “Suspended Microchannel Resonators for Biomolecular Detection,” Applied Physics Letters, vol. 83, no. 13, pp. 2698-2700, 2003.
[CrossRef] [Google Scholar] [Publisher Link]
[54] Jürgen Fritz et al., “Electronic Detection of DNA by Its Intrinsic Molecular Charge,” Proceedings of the National Academy of Sciences of the United States of America, vol. 99, no. 22, pp. 14142-14146, 2002.
[CrossRef] [Google Scholar] [Publisher Link]
[55] Petr Skládal, “Piezoelectric Quartz Crystal Sensors Applied for Bioanalytical Assays and Characterization of Affinity Interactions,” Journal of the Brazilian Chemical Society, vol. 14, no. 4, pp. 491-502, 2003.
[CrossRef] [Google Scholar] [Publisher Link]
[56] Edward S. Park, Jan Krajniak, and Hang Lu, “Packaging for Bio-Micro-Electro-Mechanical Systems (Biomems) and Microfluidic Chips,” Nano-Bio- Electronic, Photonic and MEMS Packaging, pp. 505-563, 2010.
[CrossRef] [Google Scholar] [Publisher Link]
[57] Stephen Cooper, “Distinguishing Between Linear and Exponential Cell Growth during the Division Cycle: Single-Cell Studies, Cell-Culture Studies, and the Object of Cell-Cycle Research,” Theoretical Biology and Medical Modelling, vol. 3, pp. 1-15, 2006.
[CrossRef] [Google Scholar] [Publisher Link]
[58] D. Killander, and A. Zetterberg, “Quantitative Cytochemical Studies on Interphase Growth. I. Determination of DNA, RNA and Mass Content of Age Determined Mouse Fibroblasts in Vitro and of Intercellular Variation in Generation Time,” Experimental Cell Research, vol. 38, no. 2, pp. 272-284, 1965.
[CrossRef] [Google Scholar] [Publisher Link]
[59] J.M. Mitchison, “Growth during the Cell Cycle,” International Review of Cytology, vol. 226, pp. 165-258, 2003.
[CrossRef] [Google Scholar] [Publisher Link]
[60] Robert F. Brooks, and Robert Shields, “Cell Growth, Cell Division and Cell Size Homeostasis in Swiss 3T3 Cells,” Experimental Cell Research, vol. 156, no. 1, pp. 1-6, 1985.
[CrossRef] [Google Scholar] [Publisher Link]
[61] Paul Jorgensen et al., “Systematic Identification of Pathways That Couple Cell Growth and Division in Yeast,” Science, vol. 297, no. 5580, pp. 395-400, 2002.
[CrossRef] [Google Scholar] [Publisher Link]
[62] K. Park, and R. Bashir, “MEMS-Based Resonant Sensor With Uniform Mass Sensitivity,” Transducers 2009 - 15th International Conference on Solid-State Sensors, Actuators and Microsystems, Denver, CO, USA, pp. 1956-1958, 2009.
[CrossRef] [Google Scholar] [Publisher Link]
[63] Andrea K. Bryan et al., “Measurement of Mass, Density, and Volume During the Cell Cycle of Yeast,” Proceedings of the National Academy of Sciences of the United States of America, vol. 107, no. 3, pp. 999-1004, 2010.
[CrossRef] [Google Scholar] [Publisher Link]
[64] Amit Tzur et al., “Optimizing Optical Flow Cytometry for Cell Volume-Based Sorting and Analysis,” PLoS ONE, vol. 6, no. 1, pp. 1-9, 2011.
[CrossRef] [Google Scholar] [Publisher Link]
[65] Michel Godin et al., “Using Buoyant Mass to Measure the Growth of Single Cells,” Nature Methods, vol. 7, pp. 387-390, 2010.
[CrossRef] [Google Scholar] [Publisher Link]
[66] Thomas P. Burg et al., “Weighing of Biomolecules, Single Cells and Single Nanoparticles in Fluid,” Nature, vol. 446, pp. 1066-1069, 2007.
[CrossRef] [Google Scholar] [Publisher Link]
[67] Thomas P. Burg, John E. Sader, and Scott R. Manalis, “Nonmonotonic Energy Dissipation in Microfluidic Resonators,” Physical Review Letters, vol. 102, no. 22, 2009.
[CrossRef] [Google Scholar] [Publisher Link]
[68] Amit Tzur et al., “Cell Growth and Size Homeostasis in Proliferating Animal Cells,” Science, vol. 325, pp. 167-171, 2009.
[CrossRef] [Google Scholar] [Publisher Link]
[69] Ram Datar et al., “Cantilever Sensors: Nanomechanical Tools for Diagnostics,” MRS Bulletin, vol. 34, no. 6, pp. 449-454, 2009.
[CrossRef] [Google Scholar] [Publisher Link]
[70] C.A. Savran et al., “Microfabricated Mechanical Biosensor with Inherently Differential Readout,” Applied Physics Letters, vol. 83, no. 8, pp. 1659-1661, 2003.
[CrossRef] [Google Scholar] [Publisher Link]
[71] Ran Kafri et al., “Dynamics Extracted from Fixed Cells Reveal Feedback Linking Cell Growth to Cell Cycle,” Nature, vol. 494, pp. 480- 483, 2013.
[CrossRef] [Google Scholar] [Publisher Link]
[72] Moshe Kafri et al., “Rethinking Cell Growth Models,” FEMS Yeast Research, vol. 16, no. 7, pp. 1-6, 2016.
[CrossRef] [Google Scholar] [Publisher Link]
[73] Kidong Park et al., “Resonant MEMS Mass Sensors for Measurement of Microdroplet Evaporation,” Journal of Microelectromechanical Systems, vol. 21, no. 3, pp. 702-711, 2012.
[CrossRef] [Google Scholar] [Publisher Link]