Architecture Design Rules of ‘Moons’ of Planets in our Solar System
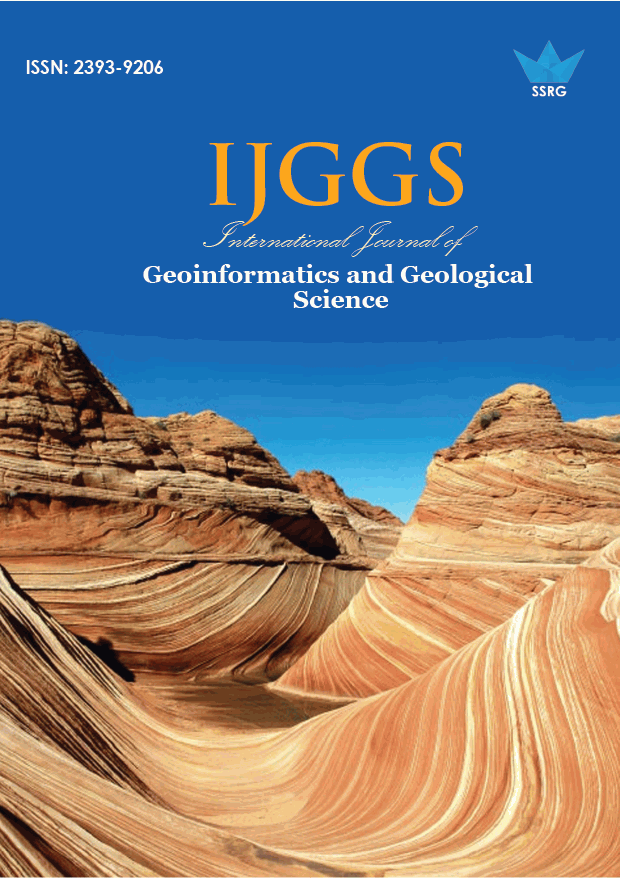
International Journal of Geoinformatics and Geological Science |
© 2024 by SSRG - IJGGS Journal |
Volume 11 Issue 1 |
Year of Publication : 2024 |
Authors : Bijay Kumar Sharma |
How to Cite?
Bijay Kumar Sharma, "Architecture Design Rules of ‘Moons’ of Planets in our Solar System," SSRG International Journal of Geoinformatics and Geological Science, vol. 11, no. 1, pp. 7-19, 2024. Crossref, https://doi.org/10.14445/23939206/IJGGS-V11I1P102
Abstract:
In our Solar System, the Sun, the Planet and its natural satellite constitute a Circular Restricted 3-Body Problem (CRTBP), which has fixed point solutions consisting of L1, L2, L3, L4 and L5. Planet, its respective natural satellite and a test particle also constitute a CRTBP and it has its corresponding 5 Lagrange Points. L4 and L5 of the Sun and Planet invariably have asteroids trapped, which constitute the Trojans of the respective Planet. There are ‘regular moons’, there are ‘irregular moons’, and there are transitional moons’ Regular moons have arisen as a by-product of the planet’s formation within the circum-planetary disk of gas and particles. The outer moons in the Hill Sphere of the respective Planet are irregular moons which are more distant and have inclined and elliptical orbits and which are captured celestial bodies from the asteroid belt from the Kuiper Belt or from the Oort’s Cloud. In the year 2000 invention of wide field Charge Coupled Device (CCD) led to a spike in the discovery of irregular moons. There are transitional ‘moons’ which lie between regular moons and irregular moons, for example Iapetus, a moon of Saturn. Finally, there are ring moons. Jupiter, Saturn, Uranus and Neptune have rings surrounding them. At the outer edge of these rings are fully formed moons. These are called ring moons. Sun and Planets (except Mercury and Venus) have captured bodies(asteroids) at L4 and L5. These captured bodies at L4 and L5 are called Trojans. Till date February 2024, Jupiter, Saturn, Uranus and Neptune have 228 irregular moons orbiting the outer four planets. The largest of these are Himalia of Jupiter, Pheobe of Saturn, Syacorax of Uranus and Triton of Neptune. Each planet has a gravitational sphere of influence known as the Hill Sphere. If the Hill Sphere is spacious enough, it captures a natural satellite.
Keywords:
Circular restricted three body problem, Trojans, Regular moons, Oort’s Cloud, Hill Sphere, Asteroid belt, KuiperBelt.
References:
[1] Dániel Apai, “Origins of Planetary Systems: Constraints and Challenges,” Earth, Moon and Planets, vol. 105, pp. 311-320, 2009.
[CrossRef] [Google Scholar] [Publisher Link]
[2] Huapei Wang et al., “Lifetime of the Solar Nebula Constraint by Meteorite Paleomagnetism,” Science, vol. 355, pp. 623-627, 2017.
[CrossRef] [Google Scholar] [Publisher Link]
[3] Bijay Kumar Sharma, “The Architectural Design Rules of Solar Systems based on the New Perspective,” Earth, Moon and Planets, vol. 108, pp. 15-37, 2011.
[CrossRef] [Google Scholar] [Publisher Link]
[4] Mark C. Wyatt, and Alan P. Jackson, “Insights into Planet Formation from Debris Disks,” Space Science Review, vol. 205, pp. 231-266, 2016.
[CrossRef] [Google Scholar] [Publisher Link]
[5] Bijay Kumar Sharma, “The Criteria for Reducing Centrally Restricted Three-Body Problem to Two-Body Problem,” International Journal of Astronomy and Astrophysics, vol. 14, pp. 1-19, 2024.
[CrossRef] [Google Scholar] [Publisher Link]
[6] Prasenjit Saha, and Scott Tremaine, “The Orbits of the Retrograde Jovian Satellites,” ICARUS, vol. 106, no. 2, pp. 549-562, 1993.
[CrossRef] [Google Scholar] [Publisher Link]
[7] Brett Gladman et al., “Discovery of 12 Satellites of Saturn Exhibiting Orbital Clustering,” Nature, vol. 412, pp. 163-188, 2001.
[CrossRef] [Google Scholar] [Publisher Link]
[8] Adrián Brunini, Mirta Gabriela Parisi, and Gonzalo Tancredi, “Constraints to Uranus’ Great Collision III the Origin of Outer Satellites,” ICARUS, vol. 159, no. 1, pp. 166-177, 2002.
[CrossRef] [Google Scholar] [Publisher Link]
[9] G. Colombo, and F.A. Franklin, “On the Formation of the Outer Satellites Groups of Jupiter,” ICARUS, vol. 16, no. 2, pp. 186-189, 1971.
[CrossRef] [Google Scholar] [Publisher Link]
[10] Sergey A. Astakhov et al., “Chaos Assisted Capture of Irregular Moons,” Nature, vol. 423, pp. 264-267, 2003.
[CrossRef] [Google Scholar] [Publisher Link]
[11] Craig B. Agnor, and Douglas P. Hamilton, “Neptune Capture of its Moon Triton in a Binary-Planet Gravitational Encounter,” Nature, 441, pp. 192-194, 2006.
[CrossRef] [Google Scholar] [Publisher Link]
[12] David Nesvorný, David Vokrouhlický, and Alessandro Morbidelli, “Capture of Irregular Satellites during Planetary Encounters,” Astronomical Journal, vol. 133, no. 5, pp. 1962-1976, 2007.
[CrossRef] [Google Scholar] [Publisher Link]
[13] David Vokrouhlický, David Nesvorný, and Harold F. Levison, “Irregular Satellites Capture Exchange Reactions,” Astronomical Journal, vol. 136, no. 4, pp. 1463-1476, 2008.
[CrossRef] [Google Scholar] [Publisher Link]
[14] H.S. Gaspar, O.C. Winter, and E. Vieira Neto, “Ïrregular Satellites of Jupiter: Capture Configurations of Binary-Asteroids,” Monthly Notices of the Royal Astronomical Society, vol. 415, no. 3, pp. 1999-2008, 2011.
[CrossRef] [Google Scholar] [Publisher Link]
[15] Catherine M. Philpott, Douglas P. Hamilton, and Craig B. Agnor, “Three-Body Capture of Irregular Satellites: Application to Jupiter,” ICARUS, vol. 208, pp. 824-836, 2010.
[CrossRef] [Google Scholar] [Publisher Link]
[16] Jay T. Bergstralh, Ellis D. Miner, and Midred Shapley Mathews, Origin of the Uranian Satellites, URANUS, University of Arizona Press, pp. 469-512, 1991.
[Google Scholar] [Publisher Link]
[17] Jonathen I. Lunine et al., “Dynamical Models of Terrestrial Planet Formation,” Advanced Science Letters, vol. 4, no. 2, pp. 325-338, 2011.
[CrossRef] [Google Scholar] [Publisher Link]
[18] David Jewitt, and Nader Haghighipour, “Irregular Satellites of the Planets: Products of Capture in the Early Solar System,” Annual Review of Astronomy and Astrophysics, vol. 45, pp. 261-295, 2007.
[CrossRef] [Google Scholar] [Publisher Link]
[19] H.F. Levison et al., “Ridge Formation and De-spinning of Iapetus via an Impact-Generated Satellite,” ICARUS, vol. 214, no. 2, pp. 773- 778, 2011.
[CrossRef] [Google Scholar] [Publisher Link]
[20] P.D. Nicholson, and L. Dones, “Planetary Rings,” Review of Geophysical, Supplement, pp. 313-327, 1991.
[21] Claudio Marazzini, “The Names of Jupiter's Satellites: from Galileo to Simon Marius,” Lettere Italiana, vol. 57, no. 3, pp. 391-407, 2005.
[Google Scholar] [Publisher Link]
[22] Katrina Miller, “An Ocean Moon Thought to be Habitable may be Oxygen Starved,” New York Times, 2024. [Online]. Available: https://www.nytimes.com/2024/03/04/science/europa-moon-oxygen.html
[23] Michael J. Malaska et al., “Organic Input to Titan’s Subsurface Ocean through Impact Cratering,” Astrobiology, vol. 24, no. 2, pp. 177- 189, 2024.
[CrossRef] [Google Scholar] [Publisher Link]
[24] M.C. Wyatt et al., “Five Steps in the Evolution from Protpplanetary Disk to Debris Disk,” Astrophysics and Space Science, vol. 357, 2015.
[CrossRef] [Google Scholar] [Publisher Link]
[25] Brenda C. Matthews, and J.J. Kavelaars, “Insight into Planet Formation from Debris Disks: I. The Solar System as an Archetype for Planetesimal,” Environmental Science Physics, vol. 205, pp. 213-230, 2016.
[CrossRef] [Google Scholar] [Publisher Link]
[26] E. Asphaug, and Andreas Reufer, “Mercury and Other Iron-Rich Planetary Bodies as Relics of Inefficient Accretion,” Nature Geoscience, vol. 7, pp. 564-568, 2014.
[CrossRef] [Google Scholar] [Publisher Link]
[27] Cedric Gillmann et al., “The Long Term Evolution of the Atmosphere of Venus: Processes and Feedback Mechanisms Interior and Exterior Exchanges,” Space Science Reviews, vol. 218, 2022.
[CrossRef] [Google Scholar] [Publisher Link]
[28] Matija Ćuk et al., “Tidal Evolution of the Moon from a High Obliquity, High Angular Momentum Earth,” Nature, vol. 539, pp. 402-406, 2016.
[CrossRef] [Google Scholar] [Publisher Link]
[29] Bijay Kumar Sharma, “Theoretical Formulation of the Phobos, Moon of Mars Rate of Altitudinal Loss,” Journal Earth and Environmental Science Research, vol. 5, no. 2, pp. 1-6, 2008.
[CrossRef] [Google Scholar] [Publisher Link]
[30] Robin Canup, and Julien Salmon, “Origin of Phobos and Deimos by the Impact of Vesta-to-Ceres Sized Body with Mars,” Science Advances, vol. 4, no. 4, 2018.
[CrossRef] [Google Scholar] [Publisher Link]
[31] Shang-Fei Liu et al., “The Formation of Jupiter’s Diluted Core by a Giant Impact,” Nature, vol. 572, pp. 355-357, 2019.
[CrossRef] [Google Scholar] [Publisher Link]
[32] Subrahmanyan Chandrasekhar, Ellipsoidal Figures of Equilibrium, The Saliman Foundation Lectures, Yale University Press, New Horizon, 1969.
[Publisher Link]
[33] J.C. Castillo-Rogez et al., “Iapetus' Geophysics: Rotation Rate, Shape, and Equatorial Ridge,” ICARUS, vol. 190, no. 1, pp. 179-202, 2007.
[CrossRef] [Google Scholar] [Publisher Link]
[34] Kurt Lambeck, and Susan Pullan, “The Lunar Fossil Bulge Hypothesis Revisited,” Physical Earth Planet International, vol. 22, pp. 29- 35, 1980.
[CrossRef] [Google Scholar] [Publisher Link]
[35] Ian Garrick-Bethell, Jack Wisdom, and Maria T. Zuber, “Evidence of a Past High Eccentricity Lunar Orbit,” Science, vol. 313, pp. 652- 655, 2006.
[CrossRef] [Google Scholar] [Publisher Link]
[36] P. Goldreich et al., “Neptune’s Story,” Science, vol. 245, no. 4917, pp. 500-504, 1989.
[CrossRef] [Google Scholar] [Publisher Link]