The Effects of Heat Treatment on Mechanical Properties and Microstructure of Wire-arc Additive Remanufacturing of AISI 4130 Steel Components
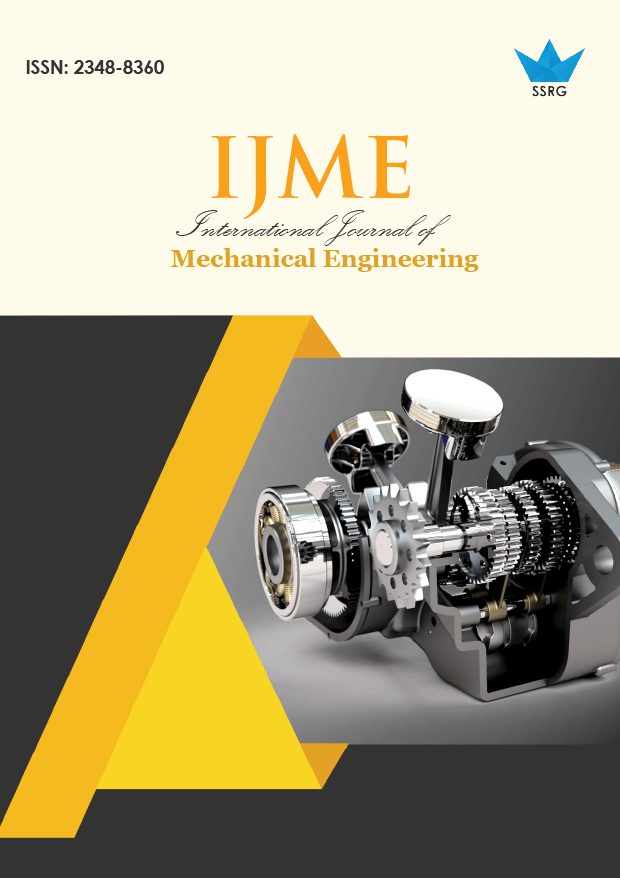
International Journal of Mechanical Engineering |
© 2024 by SSRG - IJME Journal |
Volume 11 Issue 4 |
Year of Publication : 2024 |
Authors : Talent Kachomba, James Mutua, Joshua Ngoret |
How to Cite?
Talent Kachomba, James Mutua, Joshua Ngoret, "The Effects of Heat Treatment on Mechanical Properties and Microstructure of Wire-arc Additive Remanufacturing of AISI 4130 Steel Components," SSRG International Journal of Mechanical Engineering, vol. 11, no. 4, pp. 40-55, 2024. Crossref, https://doi.org/10.14445/23488360/IJME-V11I4P105
Abstract:
This study focused on the remanufacturing of industrial components using wire-arc additive manufacturing (WAAM) and the influence of heat treatment on the mechanical properties and microstructure evolution of printed parts. The study analyzed the microstructure and mechanical properties in as-built and heat-treated conditions of the 3D printed parts. The results show that the deposited AISI 4130 steel had ferrite-pearlite phases. Microstructural analysis indicated distinct characteristics in the substrate, interface, and deposited regions. Austenitisation and tempering heat treatment caused microstructure homogenization, revealing the predominant ferrite-pearlite phase. The interface had the highest microhardness value of 180.3±6.9 HV0.3 in as-built condition. After heat treatment, the hardness improved by an average of 50.2%. The deposited region recorded a density of 7.695 g/cm3 in an as-built state. In the as-built condition, the test samples exhibited a yield strength of 172.9±2.1 MPa, but the interface sample had 162.1±5.3 MPa. The printed parts had an ultimate tensile stress of ~539.6±68.9 MPa compared to 493.3±21.8 MPa of the substrate. After heat treatment, yield strength improved by 8.9% for the printed part and decreased by 12.1% at the interface region. The ultimate strength for the samples section parallel and perpendicular to the deposition directions increased by 8.0±40.5% and the interface by 36.1%. Fractography analysis indicated that failure changed from brittle to ductile fracture after heat treatment. The findings of this study contribute to designing heat treatment schedules for industrial remanufacturing of structural and functional components using WAAM.
Keywords:
Wire-arc additive manufacturing, Remanufacturing, Heat treatment, Microstructure, Homogenization.
References:
[1] Bonny Onuike, and Amit Bandyopadhyay, “Additive Manufacturing in Repair: Influence of Processing Parameters on Properties of Inconel 718,” Materials Letters, vol. 252, pp. 256-259, 2019.
[CrossRef] [Google Scholar] [Publisher Link]
[2] Guijun Bi, and Andres Gasser, “Restoration of Nickel-Base Turbine Blade Knife-Edges with Controlled Laser Aided Additive Manufacturing,” Physics Procedia, vol. 12, pp. 402-409, 2011.
[CrossRef] [Google Scholar] [Publisher Link]
[3] Rahito, D.A. Wahab, and A.H. Azman, “Additive Manufacturing for Repair and Restoration in Remanufacturing: An Overview from Object Design and Systems Perspectives,” Processes, vol. 7, no. 1, pp. 1-22, 2019.
[CrossRef] [Google Scholar] [Publisher Link]
[4] Rahito, Dzuraidah Abd Wahab, and Abdul Hadi Azman, “Restoration of Remanufacturable Components Using Additive Manufacturing,” Proceedings of the 5th International Conference on Sustainable Design and Manufacturing, vol. 130, pp. 193-198, 2019.
[CrossRef] [Google Scholar] [Publisher Link]
[5] H. Koehler et al., “Laser Reconditioning of Crankshafts: From Lab to Application,” Physics Procedia, vol. 5, pp. 387-397, 2010.
[CrossRef] [Google Scholar] [Publisher Link]
[6] Abdollah Saboori et al., “Application of Directed Energy Deposition-Based Additive Manufacturing in Repair,” Applied Sciences, vol. 9, no. 16, pp. 1-26, 2019.
[CrossRef] [Google Scholar] [Publisher Link]
[7] R. Rumman et al., “Laser Metal Deposition and Wire Arc Additive Manufacturing of Materials: An Overview,” Archives of Metallurgy and Materials, vol. 64, no. 2, pp. 467-473, 2019.
[CrossRef] [Google Scholar] [Publisher Link]
[8] David Svetlizky et al., “Directed Energy Deposition (DED) Additive Manufacturing: Physical Characteristics, Defects, Challenges and Applications,” Materials Today, vol. 49, pp. 271-295, 2021.
[CrossRef] [Google Scholar] [Publisher Link]
[9] D.T. Sarathchandra, M.J. Davidson, and Gurusamy Visvanathan, “Parameters Effect on SS304 Beads Deposited by Wire Arc Additive Manufacturing,” Materials and Manufacturing Processes, vol. 35, no. 7, pp. 852-858, 2020.
[CrossRef] [Google Scholar] [Publisher Link]
[10] Laukik P. Raut, and Ravindra V. Taiwade, “Wire Arc Additive Manufacturing: A Comprehensive Review and Research Directions,” Journal of Materials Engineering and Performance, vol. 30, pp. 4768-4791, 2021.
[CrossRef] [Google Scholar] [Publisher Link]
[11] Muralimohan Cheepu, Chang Ik Lee, and Sang Myung Cho, “Microstructural Characteristics of Wire Arc Additive Manufacturing with Inconel 625 by Super-TIG Welding,” Transactions of the Indian Institute of Metals, vol. 73, pp. 1475-1479, 2020.
[CrossRef] [Google Scholar] [Publisher Link]
[12] Reyazul Warsi, Kashif Hasan Kazmi, and Mukesh Chandra, “Mechanical Properties of Wire and Arc Additive Manufactured Component Deposited by a CNC Controlled GMAW,” Materials Today: Proceedings, vol. 56, pp. 2818-2825, 2022.
[CrossRef] [Google Scholar] [Publisher Link]
[13] Tiago A. Rodrigues et al., “Current Status and Perspectives on Wire and Arc Additive Manufacturing (WAAM),” Materials, vol. 12, no. 7, pp. 1-41, 2019.
[CrossRef] [Google Scholar] [Publisher Link]
[14] C.R. Cunningham et al., “Invited Review Article: Strategies and Processes for High-Quality Wire Arc Additive Manufacturing,” Additive Manufacturing, vol. 22, pp. 672-686, 2018.
[CrossRef] [Google Scholar] [Publisher Link]
[15] Jeong-Hak Lee, Choon-Man Lee, and Dong-Hyeon Kim, “Repair of Damaged Parts Using Wire Arc Additive Manufacturing in Machine Tools,” Journal of Materials Research and Technology, vol. 16, pp. 13-24, 2022.
[CrossRef] [Google Scholar] [Publisher Link]
[16] T.S. Senthil et al., “Mechanical and Microstructural Characterization of Functionally Graded Inconel 825-SS316L Fabricated Using Wire Arc Additive Manufacturing,” Journal of Materials Research and Technology, vol. 15, pp. 661-669, 2021.
[CrossRef] [Google Scholar] [Publisher Link]
[17] Van Thao Le et al., “Wire and Arc Additive Manufacturing of 308L Stainless Steel Components : Optimization of Processing Parameters and Material Properties,” Engineering Science and Technology, an International Journal, vol. 24, no. 4, pp. 1015-1026, 2021.
[CrossRef] [Google Scholar] [Publisher Link]
[18] Yashwant Koli et al., “Investigations and Multi-Response Optimization of Wire Arc Additive Manufacturing Cold Metal Transfer Process Parameters for Fabrication of SS308L Samples,” Journal of Materials Engineering and Performance, vol. 32, pp. 2463-2475, 2023.
[CrossRef] [Google Scholar] [Publisher Link]
[19] Frank Meiners et al., “New Hybrid Manufacturing Routes Combining Forging and Additive Manufacturing to Efficiently Produce High-Performance Components from Ti-6Al-4V,” Procedia Manufacturing, vol. 47, pp. 261-267, 2020.
[CrossRef] [Google Scholar] [Publisher Link]
[20] Anup Kulkarni, D.K. Dwivedi, and M. Vasudevan, “Microstructure and Mechanical Properties of A-TIG Welded AISI 316L SS-Alloy 800 Dissimilar Metal Joint,” Materials Science and Engineering: A, vol. 790, 2020.
[CrossRef] [Google Scholar] [Publisher Link]
[21] Malcolm Dinovitzer et al., “Effect of Wire and Arc Additive Manufacturing (WAAM) Process Parameters on Bead Geometry and Microstructure,” Additive Manufacturing, vol. 26, pp. 138-146, 2019.
[CrossRef] [Google Scholar] [Publisher Link]
[22] Abdulaziz I. Albannai, “A Brief Review on the Common Defects in Wire Arc Additive Manufacturing,” International Journal of Current Science Research and Review, vol. 5, no. 12, pp. 4556-4576, 2022.
[CrossRef] [Google Scholar] [Publisher Link]
[23] Yufeng Xia et al., “Multi-Properties Optimization of Welding Parameters of Wire Arc Additive Manufacture in Dissimilar Joint of Iron-Based Alloy and Nickel-Based Superalloy Using Grey-Based Taguchi Method,” Proceedings of the Institution of Mechanical Engineers, Part C: Journal of Mechanical Engineering Science, vol. 235, no. 23, pp. 6984-6995, 2021.
[CrossRef] [Google Scholar] [Publisher Link]
[24] Fernando Veiga et al., “Wire Arc Additive Manufacturing Process for Topologically Optimized Aeronautical Fixtures,” 3D Printing and Additive Manufacturing, vol. 10, no. 1, pp. 23-33, 2023.
[CrossRef] [Google Scholar] [Publisher Link]
[25] Oguzhan Yilmaz, Nabil Gindy, and Jian Gao, “A Repair and Overhaul Methodology for Aeroengine Components,” Robotics and Computer-Integrated Manufacturing, vol. 26, no. 2, pp. 190-201, 2010.
[CrossRef] [Google Scholar] [Publisher Link]
[26] Yimin Zhuo et al., “Microstructure and Mechanical Properties of Wire Arc Additive Repairing Ti–5Al–2Sn–2Zr–4Mo–4Cr Titanium Alloy,” Materials Science and Technology, vol. 36, no. 15, pp. 1712-1719, 2020.
[CrossRef] [Google Scholar] [Publisher Link]
[27] Cui E. Seow et al., “Wire + Arc Additively Manufactured Inconel 718: Effect of Post-Deposition Heat Treatments on Microstructure and Tensile Properties,” Materials & Design, vol. 183, pp. 1-12, 2019.
[CrossRef] [Google Scholar] [Publisher Link]
[28] Wei You et al., “Materialometrical Approach of Predicting the Austenite Formation Temperatures,” Materials Science and Engineering: A, vol. 419, no. 1-2, pp. 276-282, 2006.
[CrossRef] [Google Scholar] [Publisher Link]
[29] Chandan Pandey et al., “Some Studies on P91 Steel and their Weldments,” Journal of Alloys and Compounds, vol. 743, pp. 332-364, 2018.
[CrossRef] [Google Scholar] [Publisher Link]
[30] O. Heidary et al., “UP-Quenched SAE 4130 Steel: Mechanical Properties Assessment and Bainite Formation,” Materials Science and Engineering: A, vol. 787, 2020.
[CrossRef] [Google Scholar] [Publisher Link]
[31] K.M. Rajan, P.U. Deshpande, and K. Narasimhan, “Effect of Heat Treatment of Preform on the Mechanical Properties of Flow Formed AISI 4130 Steel Tubes–A Theoretical and Experimental Assessment,” Journal of Materials Processing Technology, vol. 125-126, pp. 503-511, 2002.
[CrossRef] [Google Scholar] [Publisher Link]
[32] Giuseppe Casalino et al., “Experimental and Numerical Study of AISI 4130 Steel Surface Hardening by Pulsed Nd:YAG Laser,” Materials, vol. 12, no. 19, pp. 1-19, 2019.
[CrossRef] [Google Scholar] [Publisher Link]
[33] O. Heidary et al., “Texture Development during Austempering Process of an AISI 4130 Steel,” Materials Science and Engineering: A, vol. 793, 2020.
[CrossRef] [Google Scholar] [Publisher Link]
[34] Robert P. Mudge, and Nicholas R. Wald, “Laser Engineered Net Shaping Advances Additive Manufacturing and Repair,” Welding Journal-New York, vol. 86, no. 1, pp. 44-48, 2007.
[Google Scholar] [Publisher Link]
[35] Hélène Bultel, and Jean-Bernard Vogt, “Influence of Heat Treatment on Fatigue Behaviour of 4130 AISI Steel,” Procedia Engineering, vol. 2, no. 1, pp. 917-924, 2010.
[CrossRef] [Google Scholar] [Publisher Link]
[36] Do Sik Shim et al., “Effects of Pre- and Post-Repair Heat Treatments on Microstructure and Tensile Behaviors of 630 Stainless Steel Repaired by Metal Additive Manufacturing,” Journal of Materials Research and Technology, vol. 13, pp. 980-999, 2021.
[CrossRef] [Google Scholar] [Publisher Link]
[37] Wei Liu, and Deli Gao, “Hall-Petch Relation in the Fracture Strength of Matrix-Body PDC Bits,” International Journal of Refractory Metals and Hard Materials, vol. 98, 2021.
[CrossRef] [Google Scholar] [Publisher Link]
[38] M.R. Taylor, A.D. Murdock, and S.M. Evans, “High Penetration Rates and Extended Bit Life through Revolutionary Hydraulic and Mechanical Design in PDC Drill Bit Development,” SPE Drilling & Completion, vol. 14, no. 1, pp. 34-41, 1999.
[CrossRef] [Google Scholar] [Publisher Link]
[39] L.M. Smith, V.P. Perrin, and R. Delwiche, “Steel Body PDC Bit Technology Demonstrates Performance Improvements,” IADC/SPE Asia Pacific Drilling Technology, Jakarta, Indonesia, 1998.
[CrossRef] [Google Scholar] [Publisher Link]
[40] Mehran Zamani, Hamed Mirzadeh, and Hamid M. Ghasemi, “Mechanical Properties and Fracture Behavior of Intercritically Annealed AISI 4130 Chromoly Steel,” Materials Research Express, vol. 5, no. 6, 2018.
[CrossRef] [Google Scholar] [Publisher Link]
[41] ASTM E3-11, Standard Guide for Preparation of Metallographic Specimens, American Society for Testing and Materials, pp. 1-12, 2017.
[CrossRef] [Publisher Link]
[42] ASTM E384-17, Standard Test Method for Microindentation Hardness of Materials, American Society for Testing and Materials, pp. 1- 40, 2022.
[CrossRef] [Publisher Link]
[43] ASTM E8/E8M-22, Standard Test Methods for Tension Testing of Metallic, American Society for Testing and Materials, pp. 1-31, 2010.
[CrossRef] [Google Scholar] [Publisher Link]
[44] Lioudmila A. Matlakhova et al., “Phase Composition and Temperature Effect on the Dynamic Young’s Modulus, Shear Modulus, Internal Friction, and Dilatometric Changes in AISI 4130 Steel,” Crystals, vol. 13, no. 6, pp. 1-17, 2023.
[CrossRef] [Google Scholar] [Publisher Link]
[45] T. Mukherjee et al., “Spatial and Temporal Variation of Hardness of a Printed Steel Part,” Acta Materialia, vol. 209, 2021.
[CrossRef] [Google Scholar] [Publisher Link]
[46] Jiaqing Yin, Mats Hillert, and Annika Borgenstam, “Morphology of Upper and Lower Bainite with 0.7 Mass Pct C,” Metallurgical and Materials Transactions A, vol. 48, pp. 4006-4024, 2017.
[CrossRef] [Google Scholar] [Publisher Link]
[47] M. Abdelwahed et al., “Effect of Water Atomization on Properties of Type 4130 Steel Processed by L-PBF,” Materials & Design, vol. 210, pp. 1-16, 2021.
[CrossRef] [Google Scholar] [Publisher Link]
[48] William D. Callister, and Jr. David G. Rethwisch, Materials Science and Engineering, Wiley, 2008.
[Google Scholar] [Publisher Link]
[49] R. Steadman, “Materials Science,” Physics Education, vol. 5, no. 2, 1970.
[Google Scholar] [Publisher Link]
[50] P. Wang et al., “Effect of Delta Ferrite on Impact Properties of Low Carbon 13Cr-4Ni Martensitic Stainless Steel,” Materials Science and Engineering: A, vol. 527, no. 13-14, pp. 3210-3216, 2010.
[CrossRef] [Google Scholar] [Publisher Link]
[51] Adnan Çalik, “Effect of Cooling Rate on Hardness and Microstructure of AISI 1020, AISI 1040 and AISI 1060 Steels,” International Journal of Physical Sciences, vol. 4, no. 9, pp. 514-518, 2009.
[Google Scholar] [Publisher Link]
[52] Mengchao Song et al., “Effect of Cooling Rate on Microstructure Evolution and Hardness of Steel Balls,” Transactions of the Indian Institute of Metals, vol. 73, pp. 913-920, 2020.
[CrossRef] [Google Scholar] [Publisher Link]
[53] Markus Köhler, Jonas Hensel, and Klaus Dilger, “Effects of Thermal Cycling on Wire and Arc Additive Manufacturing of Al-5356 Components,” Metals, vol. 10, no. 7, pp. 1-11, 2020.
[CrossRef] [Google Scholar] [Publisher Link]
[54] Fakada Dabalo Gurmesa, and Hirpa Gelgele Lemu, “Literature Review on Thermomechanical Modelling and Analysis of Residual Stress Effects in Wire Arc Additive Manufacturing,” Metals, vol. 13, no. 3, pp. 1-24, 2023.
[CrossRef] [Google Scholar] [Publisher Link]
[55] Witold Brostow, Haley E. Hagg Lobl, and Moshe Narkis, “The Concept of Materials Brittleness and its Applications,” Polymer Bulletin, vol. 67, pp. 1697-1707, 2011.
[CrossRef] [Google Scholar] [Publisher Link]
[56] P.S. Beshenkov et al., “Stress Distribution Analysis of PDC Drill Bits by Computer Modeling,” Eurasian Mining, no. 2, pp. 25-28, 2017.
[CrossRef] [Google Scholar] [Publisher Link]
[57] Teresa Artaza et al., “Wire Arc Additive Manufacturing Ti6Al4V Aeronautical Parts Using Plasma Arc Welding: Analysis of Heat-Treatment Processes in Different Atmospheres,” Journal of Materials Research and Technology, vol. 9, no. 6, pp. 15454-15466, 2020.
[CrossRef] [Google Scholar] [Publisher Link]
[58] Yachao Wang, and Jing Shi, “Effect of Post Heat Treatment on the Microstructure and Tensile Properties of Nano TiC Particulate Reinforced Inconel 718 by Selective Laser Melting,” Journal of Manufacturing Science and Engineering, vol. 142, no. 5, pp. 1-12, 2020.
[CrossRef] [Google Scholar] [Publisher Link]
[59] V. Manivel Muralidaran et al., “Effect of Preheating Temperatures on Impact Properties of Chromoly Alloy Steel 4130 Weld Using Gas Metal Arc Welding,” International Journal of Civil Engineering and Technology, vol. 8, no. 9, pp. 319-327, 2017.
[Google Scholar] [Publisher Link]
[60] Alireza M. Haghighi, and Farhad S. Samani, “Effects of Welding Parameters, Time Interval and Preheating on Residual Stress and Distortion of Joining ST52 Stiffener Ring in an AISI 4130 Tubular Shell,” Acta Mechanica Malaysia, vol. 3, no. 1. pp. 11-15, 2020.
[CrossRef] [Google Scholar] [Publisher Link]
[61] Jian Wang et al., “A Study on Obtaining Equiaxed Prior-β Grains of Wire and Arc Additive Manufactured Ti–6Al–4V,” Materials Science and Engineering: A, vol. 772, 2020.
[CrossRef] [Google Scholar] [Publisher Link]
[62] Shusen Luo, Zesheng You, and Lei Lu, “Thickness Effect on Fracture Behavior of Columnar-Grained Cu with Preferentially Oriented Nanoscale Twins,” Journal of Materials Research, vol. 32, no. 24, pp. 4554-4562, 2017.
[CrossRef] [Google Scholar] [Publisher Link]
[63] Colin Williams et al., “Nondestructive Evaluation of Fracture Toughness in 4130 Steel Using Nonlinear Ultrasonic Testing,” Journal of Nondestructive Evaluation, vol. 41, 2022.
[CrossRef] [Google Scholar] [Publisher Link]
[64] Hanxue Cao et al., “The Stress Concentration Mechanism of Pores Affecting the Tensile Properties in Vacuum Die Casting Metals,” Materials, vol. 13, no. 13, pp. 1-14, 2020.
[CrossRef] [Google Scholar] [Publisher Link]
[65] Chandan Pandey et al., “Homogenization of P91 Weldments Using Varying Normalizing and Tempering Treatment,” Materials Science and Engineering: A, vol. 710, pp. 86-101, 2018.
[CrossRef] [Google Scholar] [Publisher Link]