Development and Characterization of Polymer Ceramic Composite for Biomedical Application
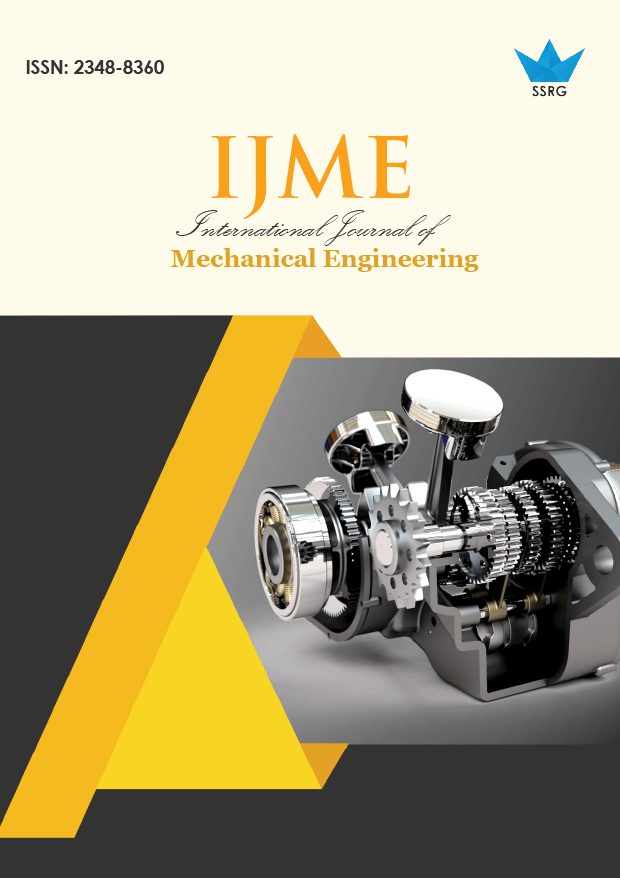
International Journal of Mechanical Engineering |
© 2024 by SSRG - IJME Journal |
Volume 11 Issue 6 |
Year of Publication : 2024 |
Authors : T. N. Aditya, P. Ravinder Reddy, P. Ramesh Babu |
How to Cite?
T. N. Aditya, P. Ravinder Reddy, P. Ramesh Babu, "Development and Characterization of Polymer Ceramic Composite for Biomedical Application," SSRG International Journal of Mechanical Engineering, vol. 11, no. 6, pp. 1-6, 2024. Crossref, https://doi.org/10.14445/23488360/IJME-V11I6P101
Abstract:
Polymer ceramic composites are finding applications in many fields, one of which is biomedical. Scaffolds act as support material for bone regeneration. Polylactic acid, a naturally degradable material which has good mechanical properties, is chosen as the polymer matrix, and 45S5 bioactive glass is chosen as ceramic filler, which is bio active. The weight proportions of 2.5,5 and 10 of filler are added to the polymer matrix and extruded to get a filament of diameter 1.75 mm. The filaments are 3d printed into cubical scaffolds. The mechanical characteristics of 3d printed composites are investigated.
Keywords:
3D printing, Composite, Scaffold, Bioactive glass, PLA.
References:
[1] Ami R. Amini, Cato T. Laurencin, and Syam P. Nukavarapu, “Bone Tissue Engineering: Recent Advances and Challenges,” Critical Reviewsin Biomedical Engineering, vol. 40, no. 5, pp. 363-408, 2012.
[CrossRef] [Google Scholar] [Publisher Link]
[2] Gabriel Fernandez de Grado et al., “Bone Substitutes: A Review of Their Characteristics, Clinical Use, and Perspectives for Large Bone Defects Management,” Journal of Tissue Engineering, vol. 9, pp. 1-18, 2018.
[CrossRef] [Google Scholar] [Publisher Link]
[3] Larry L. Hench, Ionnis D. Xynos, and Julia M. Polak, “Bioactive Glasses for in Situ Tissue Regeneration,” Journal of Biomaterials Science, Polymer Edition, vol. 15, no. 4, pp. 543-562, 2004.
[CrossRef] [Google Scholar] [Publisher Link]
[4] Aleksandra Wajda, and Maciej Sitarz, “Structural and Microstructural Comparison of Bioactive Melt-Derived and Gel-Derived Glasses from CaO-SiO2 Binary System,” Ceramics International, vol. 44, no. 8, pp. 8858-8863, 2018.
[CrossRef] [Google Scholar] [Publisher Link]
[5] Anastasiia Kashirina et al., “Biopolymers for Bone Substitutes: A Review,” Biomaterials Science, vol. 7, no. 10, pp. 3961-3983, 2019.
[CrossRef] [Google Scholar] [Publisher Link]
[6] Ganesh Narayanan et al. “Poly (Lactic Acid)-Based Biomaterials for Orthopaedic Regenerative Engineering,” Advanced Drug Delivery Reviews, vol. 107, pp. 247-276, 2016.
[CrossRef] [Google Scholar] [Publisher Link]
[7] Gurbinder Kaur et al. “Mechanical Properties of Bioactive Glasses, Ceramics, Glass-Ceramics and Composites: State-of-the-Art Review and Future Challenges,” Materials Science and Engineering: C, vol. 104, pp. 1-14, 2019.
[CrossRef] [Google Scholar] [Publisher Link]
[8] Mojtaba Mansoorianfar et al., “Cellulose-Reinforced Bioglass Composite as Flexible Bioactive Bandage to Enhance Bone Healing,” Ceramics International, vol. 47, no. 1, pp. 416-423, 2021.
[CrossRef] [Google Scholar] [Publisher Link]
[9] Seok-Jung Hong et al., “Novel Scaffolds of Collagen with Bioactive Nanofiller for the Osteogenic Stimulation of Bone Marrow Stromal Cells,” Journal of Biomaterials Applications, vol.24, no. 8, pp. 733-750, 2010.
[CrossRef] [Google Scholar] [Publisher Link]
[10] Timo J. Lehtonen, Jukka U. Tuominen, and Elina Hiekkanen, “Resorbable Composites with Bioresorbable Glass Fibers for LoadBearing Applications, in Vitro Degradation and Degradation Mechanism,” Acta Biomaterialia, vol. 9, no. 1, pp. 4868-4877, 2013.
[CrossRef] [Google Scholar] [Publisher Link]
[11] J. Russias et al., “Fabrication and in Vitro Characterization of Three-Dimensional Organic/Inorganic Scaffolds by Robocasting,” Journal of Biomedical Materials Research, vol. 83, no. 2, pp. 434-445, 2007.
[CrossRef] [Google Scholar] [Publisher Link]
[12] V. Cannillo et al., “Production of Bioglass® 45S5-Polycaprolactone Composite Scaffolds via Salt-Leaching,” Composite Structures, vol. 92, no. 8, pp. 1823-1832, 2010.
[CrossRef] [Google Scholar] [Publisher Link]
[13] Superb K. Misra et al. “Fabrication and Characterization of Biodegradable Poly(3-Hydroxybutyrate) Composite Containing Bioglass,” Biomacromolecules, vol. 8, no. 7, pp. 2112-2119, 2007.
[CrossRef] [Google Scholar] [Publisher Link]
[14] Hamidreza Pirayesh, and John A. Nychka, “Sol-Gel Synthesis of Bioactive Glass-Ceramic 45S5 and Its in Vitro Dissolution and Mineralization Behavior,” Journal of the American Ceramic Society, vol. 96, no. 5, pp. 1643-1650, 2013.
[CrossRef] [Google Scholar] [Publisher Link]
[15] E. Nejati, H. Mirzadeh, and M. Zandi, “Synthesis and Characterization of Nano-Hydroxyapatite Rods/Poly(L-Lactide Acid) Composite Scaffolds for Bone Tissue Engineering,”Composites Part A: Applied Science and Manufacturing, vol. 39, no. 10, pp. 1589-1596, 2008
[CrossRef] [Google Scholar] [Publisher Link]
[16] Talita Martins et al., “Novel 3D Composites with Highly Flexible Behavior Based on Chitosan and Bioactive Glass for Biomedical Applications,” Materials Chemistry and Physics, vol. 189, pp. 1-11, 2017.
[CrossRef] [Google Scholar] [Publisher Link]
[17] Filipe V. Ferreira et al., “Nanocellulose/Bioactive Glass Cryogelsas Scaffolds for Bone Regeneration,” Nanoscale, vol. 11, pp. 1984219849, 2019.
[CrossRef] [Google Scholar] [Publisher Link]
[18] Dibakar Mondal et al., “Porous and Biodegradable Polycaprolactone-Borophosphosilicate Hybrid Scaffolds for Osteoblast Infiltration and Stem Cell Differentiation,” Journal of the Mechanical Behavior of Biomedical Materials, vol. 92, pp. 162-171, 2019.
[CrossRef] [Google Scholar] [Publisher Link]
[19] Lutz-Christian Gerhardt et al., “The Pro-Angiogenic Properties of Multi-Functional Bioactive Glass Composite Scaffolds,” Biomaterials, vol. 32, no. 17, pp. 4096-4108, 2011.
[CrossRef] [Google Scholar] [Publisher Link]
[20] Swee Hin Teoh et al., “Three-Dimensional Bioresorbable Scaffolds for Tissue Engineering Applications,” U.S. Patent 7968026B1, pp. 1-20, 2011.
[Google Scholar] [Publisher Link]
[21] S. Harsha Rao et al., “Natural and Synthetic Polymers/Bioceramics/Bioactive Compounds-Mediated Cell Signalling in Bone Tissue Engineering,” International Journal of Biological Macromolecules, vol. 110, pp. 88-96, 2018.
[CrossRef] [Google Scholar] [Publisher Link]
[22] Rachele Sergi, Devis Bellucci, and Valeria Cannillo, “A Review of Bioactive Glass/Natural Polymer Composites: State of the Art,” Materials, vol. 13, no. 23, pp. 1-38, 2020.
[CrossRef] [Google Scholar] [Publisher Link]
[23] V. Maquet et al., “Porous Poly (α-Hydroxyacid)/Bioglass®Composite Scaffolds for Bone Tissue Engineering. I: Preparation and in Vitro Characterisation,”Biomaterials, vol. 25, no. 18, pp. 4185-4194, 2004.
[CrossRef] [Google Scholar] [Publisher Link]
[24] Habib Belaida et al., “Development of New Biocompatible 3D Printed Graphene Oxide-Based Scaffolds,” Materials Science and Engineering: C, vol. 110, pp. 1-10, 2020.
[CrossRef] [Google Scholar] [Publisher Link]
[25] Socrates Radhakrishnan et al., “Fabrication of 3D Printed Antimicrobial Polycaprolactone Scaffolds for Tissue Engineering Applications,” Materials Science and Engineering: C, vol. 118, pp. 111525-111547, 2021.
[CrossRef] [Google Scholar] [Publisher Link]
[26] Henna Niiranen et al., “In Vitro and in Vivo Behaviorof Self-Reinforced Bioabsorbable Polymer and Self-Reinforced Bioabsorbable Polymer/Bioactive Glass Composites,” Journal of Biomedical Materials Research, vol. 69A, no. 4, pp. 699-708, 2004.
[CrossRef] [Google Scholar] [Publisher Link]
[27] Sandra Hofmann, and Marcos Garcia-Fuentes, Bioactive Scaffolds for the Controlled Formation of Complex Skeletal Tissues, Regenerative Medicine and Tissue Engineering: Cells and Biomaterials, pp. 393-432, 2011.
[Google Scholar] [Publisher Link]
[28] Dong Choon Sin et al., “Polyurethane (PU) Scaffolds Prepared by Solvent Casting/Particulate Leaching (SCPL) Combined with Centrifugation,” Materials Science and Engineering: C, vol. 30, no. 1, pp. 78-85, 2010.
[CrossRef] [Google Scholar] [Publisher Link]
[29] Andreas Pfister et al., “Polymer Ionomers for Rapid Prototyping and Rapid Manufacturing by Means of 3D Printing,” Macromolecular Materials and Engineering, vol. 290, no. 2, pp. 99-113, 2005.
[CrossRef] [Google Scholar] [Publisher Link]
[30] Russell A. Giordano et al., “Mechanical Properties of Dense Polylactic Acid Structures Fabricated by Three Dimensional Printing,” Journal of Biomaterials Science, Polymer Edition, vol. 8, no. 1, pp. 63-75, 1997.
[CrossRef] [Google Scholar] [Publisher Link]
[31] C.Y. Xu et al., “Aligned Biodegradable Nanofibrous Structure: A Potential Scaffold for Blood Vessel Engineering,” Biomaterials, vol. 25, no. 5, pp. 877-886, 2004. [CrossRef] [Google Scholar] [Publisher Link]
[32] Helen H. Lu et al., “Three-Dimensional, Bioactive, Biodegradable, Polymer-Bioactive Glass Composite Scaffolds with Improved Mechanical Properties Support Collagen Synthesis and Mineralization of Human Osteoblast-Like Cells in Vitro,” Journal of Biomedical Materials Research, vol. 64A, no. 3, pp. 465-474, 2003.
[CrossRef] [Google Scholar] [Publisher Link]
[33] B.P. Chan, and K.W. Leong, “Scaffolding in Tissue Engineering: General Approaches and Tissue-Specific Considerations,” European Spine Journal, vol. 17, pp. 467-479, 2008.
[CrossRef] [Google Scholar] [Publisher Link]