Aerodynamic Assessment of Flying Wing UAV and Impact of Dimples on its Performance
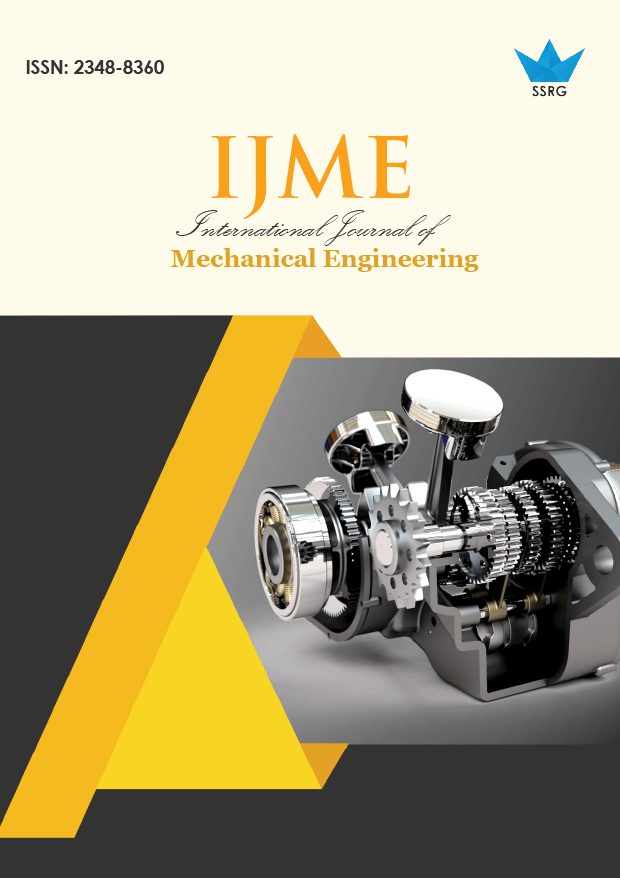
International Journal of Mechanical Engineering |
© 2024 by SSRG - IJME Journal |
Volume 11 Issue 7 |
Year of Publication : 2024 |
Authors : Sanket V. Kalgutkar, P. Booma Devi |
How to Cite?
Sanket V. Kalgutkar, P. Booma Devi, "Aerodynamic Assessment of Flying Wing UAV and Impact of Dimples on its Performance," SSRG International Journal of Mechanical Engineering, vol. 11, no. 7, pp. 98-118, 2024. Crossref, https://doi.org/10.14445/23488360/IJME-V11I7P109
Abstract:
Unmanned Aerial Vehicles (UAVs) stand out as of late because of their different applications in observation, ethereal planning, and information assortment. Aerodynamic productivity is pivotal in boosting UAV execution, especially for flying wing setups. One promising way to improve aerodynamic performance is using dimples on the UAV's wing surface, which alters the flow behavior and diminishes drag. This study plans to examine the effect of different dimple shapes on the aerodynamic characteristics of a flying wing UAV. This paper conducts a comprehensive numerical analysis using Computational Fluid Dynamics (CFD) simulations. The flying wing UAV model is exposed to various dimple arrangements, including round, square, and octagon shapes, put decisively along the wing surface. The CFD simulations utilize the SST k-w turbulence model to capture the flow features accurately. The aerodynamic assessment of each dimple shape is assessed by inspecting essential boundaries like lift coefficient, drag coefficient, and the coefficient of moment for UAV. Preliminary results indicate that dimples significantly affect the aerodynamic behavior of the flying wing UAV. Dimple shape prominently affects drag decrease, stream strength, and, by and large, lift-to-drag ratio. A similar examination gives experiences into the benefits and burdens related to various dimple shapes, aiding the plan streamlining process for flying wing UAVs. The optimized dimple shape recognized through this exploration might upgrade the UAV's endurance, range, and payload limit, making it a resource in applications requiring delayed or broadened flight activities.
Keywords:
Flying wing, UAV, CFD, Dimples, Aerodynamic.
References:
[1] M.A. Karthik et al., “Design and CFD Analysis of a Fixed Wing for an Unmanned Aerial Vehicle,” International Journal of Latest Engineering Research and Applications, vol. 2, no. 7, pp. 77-85, 2017.
[Google Scholar] [Publisher Link]
[2] Diego Armando Mejía Bugallo et al., “Design and Aerodynamic Analysis of an Unmanned Aerial Vehicle with A Fixed-Wing,” Journal of Archaeology of Egypt/Egyptology, vol. 19, no. 2, pp. 1-20, 2022.
[Google Scholar] [Publisher Link]
[3] Samuel Merryisha, and Parvathy Rajendran, “Experimental and CFD Analysis of Surface Modifiers on Aircraft Wing: A Review,” CFD Letters, vol. 11, no. 10, pp. 46-56, 2019.
[Google Scholar] [Publisher Link]
[4] V. Soundharya et al., “Aerodynamic Analysis of Dimple Effect on Airfoil,” International Journal of Engineering and Advanced Technology, vol. 5, no. 4, pp. 207-211, 2016.
[Google Scholar] [Publisher Link]
[5] Rubiat Mustak, Nizam Uddin, and Mohammad Mashud, “Effect of Different Shaped Dimples on Airfoils,” Proceeding of the International Conference on Mechanical Engineering and Renewable Energy, Chittagong, Bangladesh, pp. 1-4, 2015.
[Google Scholar] [Publisher Link]
[6] C. Ramprasadh, and V. Devanandh, “A CFD Study on Leading Edge Wing Surface Modification of a Low Aspect Ratio Flying Wing to Improve Lift Performance,” International Journal of Micro Air Vehicles, vol. 7, no. 3, pp. 361-373, 2015.
[CrossRef] [Google Scholar] [Publisher Link]
[7] T.S. Mahesh Babu et al., “Computational Analysis of Different Shapes of Dimple on Wing,” Applied Mechanics and Materials, vol. 766-767, pp. 1061-1069, 2015.
[CrossRef] [Google Scholar] [Publisher Link]
[8] E. Livya, G. Anitha, P. Valli, “Aerodynamic Analysis of Dimple Effect on Aircraft Wing,” International Journal of Mechanical, Aerospace, Industrial, Mechatronic and Manufacturing Engineering, vol. 9, no. 2, pp. 1-4, 2015.
[Google Scholar]
[9] Andrei Vladimir Popov, Ruxandra Mihaela Botez, and Michel Labib, “Transition Point Detection from the Surface Pressure Distribution for Controller Design,” Journal of Aircraft, vol. 45, no. 1, pp. 23-28, 2008.
[CrossRef] [Google Scholar] [Publisher Link]
[10] Sujit Roy et al., “Flow Control of a Wind-Turbine Airfoil with a Leading-Edge Spherical Dimple,” International Journal of Green Energy, vol. 20, no. 12, pp. 1307-1325, 2023.
[CrossRef] [Google Scholar] [Publisher Link]
[11] Long Wang et al., “Numerical Study of the Ratio of Depth-to-Print Diameter on the Performance and Flow Characteristics for a Dimpled, Highly Loaded Compressor Cascade,” Aerospace, vol. 9, no. 8, pp. 1-21, 2022.
[CrossRef] [Google Scholar] [Publisher Link]
[12] Ira H. Abbott, Albert E. Von Doenhoff, and Louis S. Stivers, “Summary of Low-Speed Airfoil Data,” National Advisory Committee for Aeronautics, NASA Technical Report, no. 824, pp. 1-261, 1945.
[Google Scholar] [Publisher Link]
[13] David C. Wilcox, “Formulation of the k-ω Turbulence Model Revisited,” AIAA Journal, vol. 46, no. 11, pp. 2823-2838, 2008.
[CrossRef] [Google Scholar] [Publisher Link]
[14] David C. Wilcox, Turbulence Modeling for CFD, DCW Industries, Incorporated, pp. 1-460, 1993.
[Google Scholar] [Publisher Link]
[15] Richard H. Pletcher, Dale Anderson, and John C. Tannehill, Computational Fluid Mechanics and Heat Transfer, 3rd ed., Taylor & Francis, pp. 1-753, 2013.
[Google Scholar] [Publisher Link]
[16] Roger E. Bilstein, “Introduction to Flight: Its Engineering and History,” Technology and Culture, vol. 21, no. 3, pp. 524-525, 1980.
[Google Scholar] [Publisher Link]
[17] Lenonard E. Schwer, “Is your Mesh Refined Enough? Estimating Discretization Error Using GCI,” 7 th LS-Dyna Anwenderforum, pp. 1-10.
[Google Scholar] [Publisher Link]
[18] Muhamad Hasfanizam Mat Yazik et al., “Computational Study on Aerodynamic Characteristics and Behaviour of S5010 Airfoil,” Journal of Advanced Research in Fluid Mechanics and Thermal Sciences, vol. 66, no. 1, pp. 42-52, 2020.
[Google Scholar] [Publisher Link]
[19] Ira H. Abbott, and A.E. Von Doenhoff, Theory of Wing Sections: Including a Summary of Airfoil Data, Dover Publications, pp. 1-704, 2012.
[Google Scholar] [Publisher Link]